1. INTRODUCTION
Oral diseases are among the most common noncommunicable diseases in the world which relate to serious health issues and economic burdens. According to The Global Burden of Disease Study (2019), it was estimated that 3.5 billion people suffering from oral diseases around the world. Among these, 2.3 billion people had permanently damaged their teeth in form of caries and tooth decay, and no less than 530 million children suffer from caries of their primary teeth [1]. Oral diseases are closely related to sugar consumption, tobacco, and alcohol abuse as well as poor oral hygiene habits. Cariogenic bacteria, especially the mutans streptococci group (S. mutans, S. sobrinus…) can attach onto tooth surfaces and produce biofilm in the presence of sugars to initiate dental caries. As the caries progress, the metabolisms of the microbial community in biofilms reduce the pH and induce the demineralization process that causes unreversible damage to the enamel layer and finally lead to tooth decay. The levels of mutans streptococci in plaque and saliva are also the reliable risk indicator for early childhood caries [2, 3].
Dental caries and tooth decay can be prevented by maintaining oral hygiene. Nowadays, besides fluoride therapies, the use of probiotics for the replacement or displacement of cariogenic bacteria in the oral cavity is a promising approach in prevention of caries. Probiotics are defined by FAO/WHO (2001) as living microorganisms that, when administered in adequate amounts, confer health benefits to the host. Most of probiotics bacteria used today are Lactic acid bacteria (LAB) and are belong to the genera Lactobacillus or Bifidobacterium [4]. Recent studies found that Lactobacillus spp. exhibiting potential properties that could make them become the promising tool to fight against S. mutans and their relating diseases. Many of Lactobacillus spp. such as L. paracasei, L. rhamnosus, L. lactis, L. fermentum, L. reuteri, L. plantarum… were found to show antagonistic activity angainst S. mutans by competing for adherence sites and nutrients; producing sort of antimicrobial secondary metabolites to inhibit S. mutans growth; and down regulating responsible genes in biofilm formation [5-14]. Chewing gum containing L. reuteri ATCC 55730 and L. reuteri ATCC PTA 5289 showed a statistically significant reduction (p < 0.05) of salivary mutans streptococci after 3 weeks of administration [15]. Also, the use of chewing gum containing these two strains reduced gingival inflammation and the levels of selected inflammatory mediators in the gingival crevicular fluid after 2 weeks [16]. Today, many chewing gum products containing probiotics can be found in the marketplace for the prevention of dental caries, improving oral health, and fighting bad breath. However, scientific papers about the preparation of probiotic chewing gum are hard to find throughout the literature, and to our knowledge, there is no paper related to this field of research was published yet in Vietnam.
From the previous study on screening of probiotics that antagonized S. mutans, L. brevis NB10 was among the most promising strains. Besides showing good in vitro effect on inhibiting the growth and biofilm formation of S. mutans, this strain meets the requirements of FAO/WHO on probiotic characteristics such as high tolerance to artificial gastrointestinal conditions and showing no risk of transferring antibiotic resistance genes [17]. In addition, being isolated from human saliva and showing high tolerance to salivary amylase, L. brevis NB10 is predicted to be able to last long in the oral cavity. L. brevis N10 is also a non-sucrose fermenter so the use of this strain would not increase the acid load in the oral cavity compared to typical Lactobacilli. For all of these reasons, it is considered that L. brevis NB10 is a good candidate to be used in chewing gum products for the prevention of S. mutans-related diseases. The current study aims to formulate the chewing gum tablet containing at least 107 CFU/tablet of L. brevis NB10. To achieve this, firstly, L. brevis NB10 freeze-drying powder was formulated. Low cariogenic cryoprotective agents were selected and screened to form a suitable protective medium that excerts a high bacterial survival rate after freeze-drying and during long-term storage. Next, chewing gum tablets were prepared from L. brevis NB10 freeze-dried powder using the direct compression method. The gum formulation was optimized for a high bacterial survival rate and good organoleptic properties. The result of this study would provide fundamentals in applying this beneficial bacteria for the prevention of dental diseases relating to S. mutans.
2. MATERIALS AND METHOD
HIG04 gum base (Health in Gum ®) was purchased from Cafosa (Barcelona, Spain). De Man, Rogosa and Sharpe (MRS) agar and MRS broth were purchased from Merck Millipore (USA). Skim milk powder was purchased from The A2 Milk Company (Australia) Pty Ltd.. All other excipients used in formulation of freeze-dried powder and chewing gum were of pharmaceutical grade. All reagents used in the analyses were of analytical grade.
L. brevis NB10 stored in -80 °C glycerol stock was thawed and streaked onto MRS agar, incubated in 24 h at 37 °C, 5% CO2. Transfer single colony from this plate into 25 ml of MRS broth and incubate in 24 h at 37 °C, 5% CO2. Finally, transfer bacteria from this broth into 250 ml of MRS broth and incubate in 24 h at 37 °C, 5% CO2. Collect bacterial cell by centrifuge at 10.000g, 4 °C for 5 min, wash the cell twice by 20 ml of cold 0.9% NaCl. Resuspend bacterial pellet into 0.9% NaCl solution to make bacterial suspension at 2 x109 CFU/ml.
The number of viable L. brevis NB10 in samples (bacterial suspension, freeze-dried powder, or chewing gum) was determined by the spread plate method. Briefly, ten-fold serial dilutions were made from 1 ml of bacterial suspension or 1 gram of examined powder, or 1 tablet of chewing gum. Then, transfer and spread 0.1 ml of each dilution onto three dishes of MRS agar, incubate these dishes in 24 h at 37 °C, 5% CO2. Select and calculate the mean colony-forming unit (CFU) from the plates corresponding to a given dilution that shows the number of colonies between 30 – 300. The quantity of viable L. brevis NB10 in samples was calculated as:
(CFU/ml or CFU/g or CFU/tablet)
Wherein, N̅ is the mean CFU from the plates of given dilution; d is the dilution factor
For freeze-dried powders, three samples will be taken to enumerate viable bacteria and calculate the average value.
To quickly estimate the viable amount of L. brevis NB10 by OD600, a series of bacterial suspensions with OD600 value from 0.1 to 2.5 was prepared using colonies from overnight MRS agar plate. Then, the logarithm of viable cell concentration (log CFU/ml) from these suspensions was determined by the spread plate method and plotted versus their OD600 values. As a result, the linear regression equation between OD600 and Log CFU/ml was made as: ŷ = 2,579x + 5,014 (R2 > 0,9994, data not shown). Therefore, the cell density of bacterial suspensions will be adjusted appropriately based on OD600 using this equation before mixing with cryoprotectants to make freeze-dried powder.
To choose the most suitable protective agents that providing the best survival rate of S. brevis NB10 after freeze-drying as well as during storage, protectants such as sorbitol, mannitol, xylitol, lactose, maltodextrin, skim milk, and potassium ascorbate were used to formulate the L. brevis NB10 freeze-dried powders. Each agent was prepared as 20% solution and autoclaved at 115 °C for 15 minutes before use, except potassium ascorbate was sterilized through 0.22 μm filter. These protective agents were used as single agent or in mixture with other agents to form different protective mediums. Then, L. brevis NB10 suspension at 2 × 109 CFU/ml was mixed with each protective medium at a ratio 1:1 to make the pre-freeze-dried samples. Determine the amount of L. brevis NB10 (CFU/ml) in these samples before drying (Q1).
Pre-freeze-dried samples were kept at -20 °C; for 4 hours then at -80 °C; for 4 hours. Next, transfer these samples to the freeze-dryer (FreeZone® 4.5L, Labconco, USA). The condenser temperature was set at -50 °C, the drying chamber was set at -20 °C;, 0.15 mbar for 16 hrs and then -10 °C; for 6 hrs. The freeze-dried powder was collected and stored in PE bag with moisture absorber. Determine the amount of L. brevis NB10 (CFU/g) in these powders after drying (Q2). The survival rate (S1) of L. brevis NB10 after freeze-drying corresponding to a given protective medium was calculated as:
Wherein, V1 is the total volume of the sample before freeze-drying; M2 is the total weight of the sample after freeze-drying.
To choose the most suitable protective agents that providing the best stability of S. brevis during storage, the freeze-dried powders were stored at 5 °C ± 3 °C or at 25 °C ± 2 °C, RH 60%. After certain periods, examine for the change in appearance of these powder. Also, the amount of L. brevis NB10 (CFU/g) in these powders was determined (Q3), and the survival rate (S2) of L. brevis NB10 during storage was calculated as:
The basic formulation of the chewing gum containing L. brevis NB10 was used as showing in Table 1. The chewing gum was prepared by direct compression method to prevent the exposure of bacteria to water, solvents or moisture during granulation as well as to reduce the preparation time thus increasing the survival rate of L. brevis NB10 after formulation. Briefly, the freeze-dried powder of L. brevis NB10 was mixed with xylitol and strawberry flavor powder, while HIG04® was mixed with aerosil, and glycerol. Next, these mixtures were blended, then mixed with lubricants. The gum tablet was made using rotary tablet machine with 15 mm dice equipped (CIP - 8. STN. O. Lab Press, India). The compression was operated manually at the speed 7 rpm. Also, all the preparation process was taken place in 20 °C and 50% RH room.
To determine the appropriate amount of each composition, series of formulations based on the basic formulation were made in which individual excipient was changed at a time to examine the effect of its on the product properties. The gum tablet properties were evaluated by the following tests.
Choose 20 random gum tablets from each batch and observe each one by the naked eye for shape, color, surface roughness, and appearance uniformity between tablets. Evaluate of weight variant according to the Vietnamese Pharmacopoeia V. No more than 2 units have the mass that are outside the limits of 95–105% of the average mass, and no tablet has the weight that is outside the limits of 90–110% of the average mass.
The amount of L. brevis NB10 (CFU/g) before and after tablet compression was determined, and the survival rate (S3) of L. brevis NB10 was calculated as:
Wherein, Q4 is the amount of L. brevis NB10 (CFU/g) in the semi-finished powder before compression; Q5 is the amount of L. brevis NB10 (CFU/tablet) in the gum tablet right after compression; mt is the weight of gum tablet.
For the content uniformity test, count the number of viable L. brevis NB10 of 10 random gum tablets from each batch. No more than one unit has the viable bacterial number that is outside the limit of 80 – 120% of average viable bacterial number, and no unit has the viable bacterial number that is outside the limit of 60 – 140% of average viable bacterial number.
Organoleptic properties of the gum tablet include chewable mass, sweetness, flavor (intense and lasting time), and softness were evaluated by 6 healthy volunteers. After signing informed consent form, each volunteer was asked to chew a gum tablet for 10 seconds, then spat out and give a score according to the product properties assessment scorecard (Table 2). The chewing gums used in these assessments were made from freeze-dried powder without L. brevis NB10.
3. RESULTS
Firstly, cryoprotectant agents were used individually to make pre-freeze-dried samples. The concentration of each agent used in pre-freeze-dried sample was 10%. The total volume of each pre-freeze-dried sample was 100 ml and the initial amount of bacteria was adjusted to approx. 109 CFU/ml. The appearance, moisture and the bacterial survival rate of each corresponding freeze-dried powder was shown (Table 3).
From this result, lactose, sorbitol and xylitol were not able to protect L. brevis NB10 during freeze-drying process. The bacterial survival rates in freeze-dried powder using these agents were lower than 10%. Also, these powders were not suitable to use in gum preparation due to rapid moisture absorbing as well as hard to grind into fine particle.
Mannitol, maltodextrin, and skim milk showed promising ability in preserving L. brevis NB10 during freeze-drying process with bacterial survival rates higher than 20%. Hence, these agents were chosen and use in the mixture to formulate the protective medium for freeze-drying of L. brevis NB10 (Table 4).
According to the results, the combination of mannitol or maltodextrin with skim milk improved the survival rate of L. brevis NB10 after the freeze-drying process, wherein three combinations showing the highest bacteria protection were Man: SM (3:7); Mal: SM (3:7) and Mal: SM (5:5) with the bacterial survival rate of 53.49%; 48.43% and 62.21%, respectively. Hence, these three combinations were selected to adjust the use amount to further improve bacterial survival (Table 5).
Of all the combinations, the three showing the best L. brevis NB10 protection ability were Man:SM (3:7) at 10%, Mal:SM (3:7) at 10%, and Mal:SM (5:5) at 12%. Next, the stability of L. brevis NB10 in these three freeze-dried when stored at room temperature was examined. The amount of live L. brevis NB10 in these powders during 75 days of storage were shown (Figure 1)
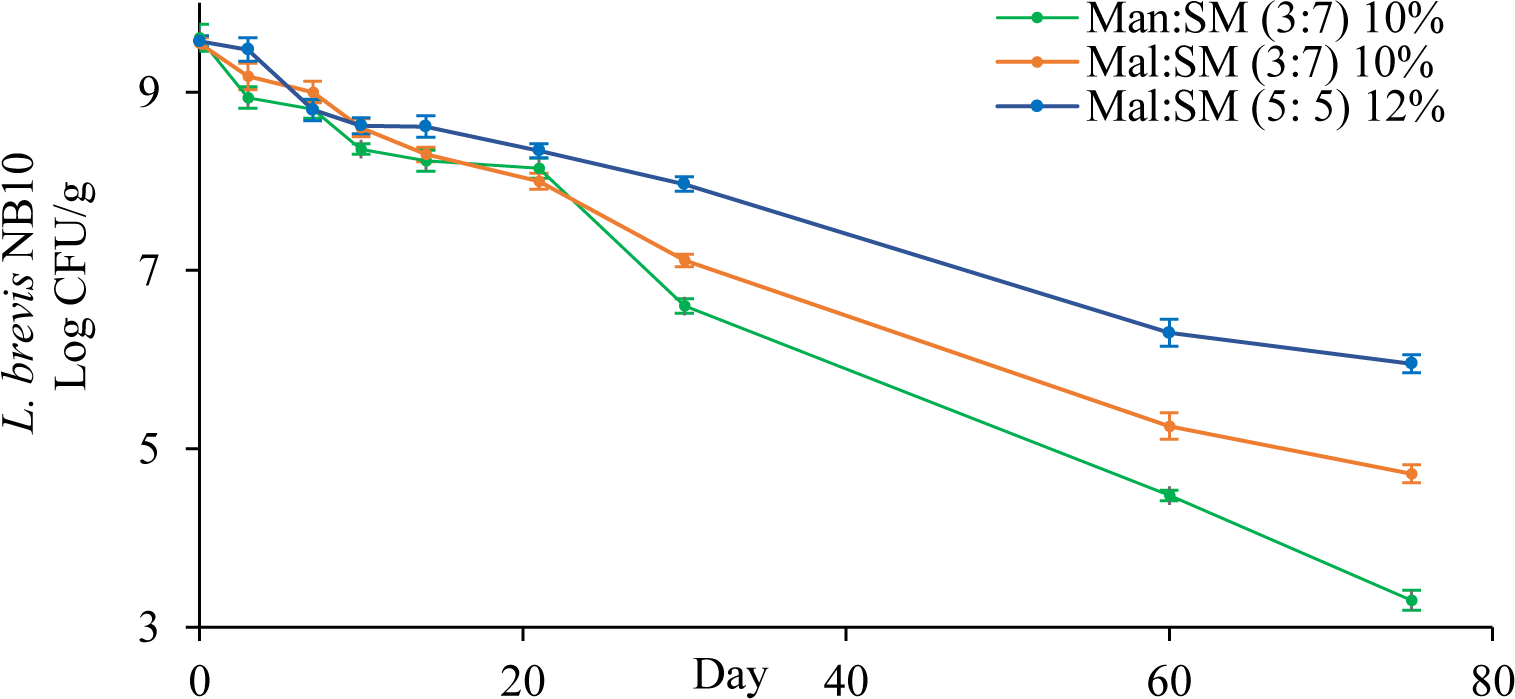
The amount of live L. brevis is gradually decreased in all tested powders during storage time, wherein, the combination of Mal:SM (5:5) at 12% (w/v) provided the highest bacterial stability. Hence, the combination of Mal:SM (5:5) at 12% (w/v) was chosen to prepare L. brevis NB10 freeze-dried powder. Next, potassium ascorbate was added to the freeze-dried formulation to increase L. brevis NB10 stability over time. The properties of these freeze-dried powders were shown in Table 6.
The addition of 0.25% - 0.5% of potassium ascorbate slightly increased the survival rate of L. brevis NB10 during the freeze-drying process (p < 0.05). However, the higher the amount of potassium ascorbate was, the darker the color of freeze-dried powders was. Also, when adding 1% of potassium ascorbate to the formulation, the freeze-dried product could not be ground into a fine powder which is not suitable to use as material in gum formulation. Hence, the freeze-dried formulations containing 0.25% and 0.5% of potassium ascorbate were selected to study the bacterial stability at room temperature (Figure 2).
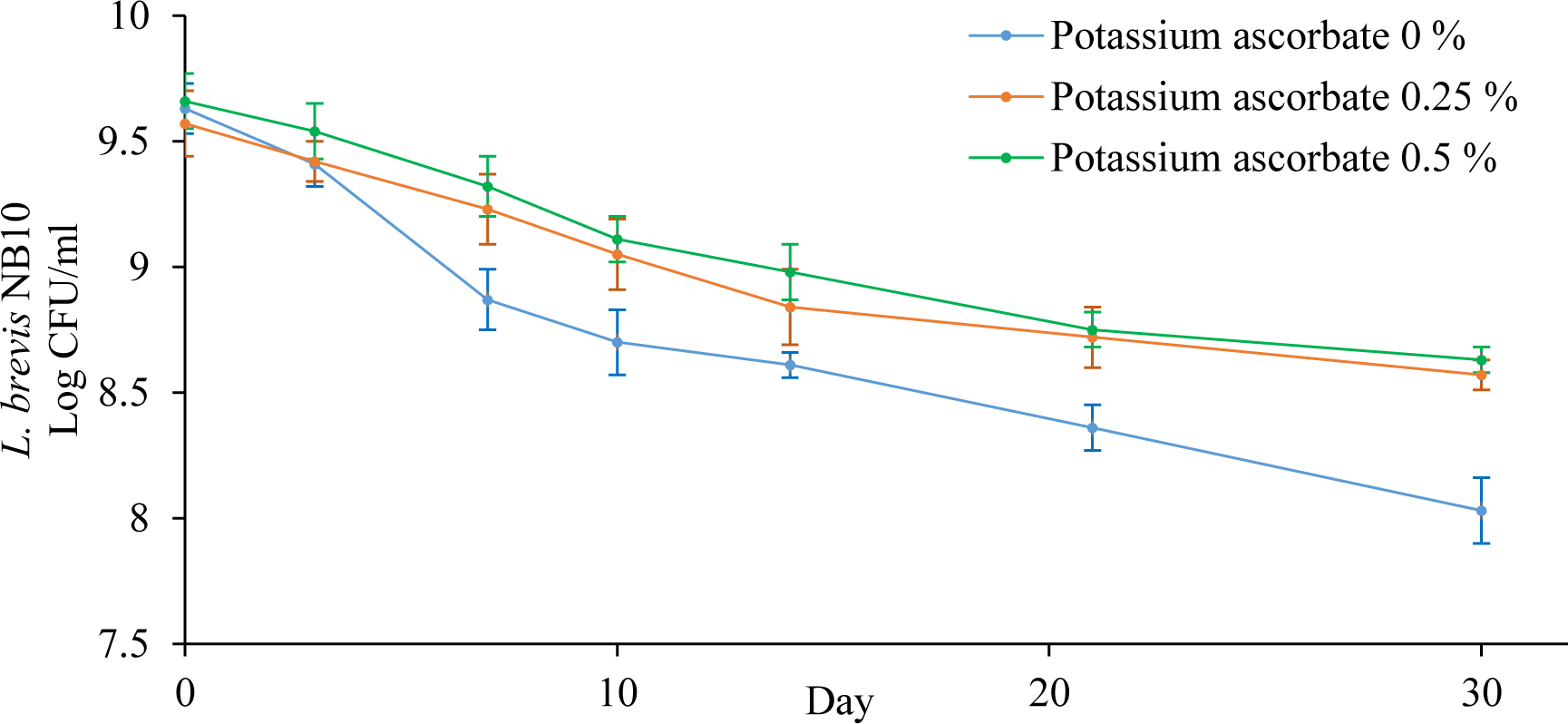
Potassium ascorbate showed a positive effect on the L. brevis NB10 survival in 30 days. However, there was no significant difference in the protective effect of potassium ascorbate at the concentrations of 0.25% or 0.5%. Thus, the optimal formulation for preparing freeze-dried L. brevis NB10 consisted of 6% maltodextrin, 6% skim milk, and 0.25% potassium ascorbate (Table 7).
No. | Composition | Amount |
---|---|---|
1 | L. brevis NB10 | 1 x 1012 CFU |
2 | Sodium chloride | 3.15 g |
3 | Potassium ascorbate | 2.5 g |
4 | Maltodextrin | 60.0 g |
5 | Skim milk | 60.0 g |
6 | Water | To 1000 ml |
To evaluate the consistence of freeze-drying formulation and preparation process, 3 lots of L. brevis NB10 freeze-dried powders were made. The total volume of each pre-freeze-dried sample from each lot was 500 ml. The properties of freeze-dried powders from these three lots were shown in Table 8.
There was no significant difference in the appearance, moisture content, and bacterial survival rate between the three studied lots. Therefore, the formulation and preparation process of L. brevis NB10 powder was consistent.
The number of viable L. brevis NB10 decreased over time when stored at 5 °C ± 3 °C (Figure 3). However, after 6 months of storage, the number of live bacteria in all 3 lots remained higher than 109 CFU/g. Also, there were no significant differences in the bacterial stability between the three studied lots (p < 0.05). Therefore, L. brevis NB10 freeze-dried powders were stable at 5 °C ± 3 °C for at least 6 months.
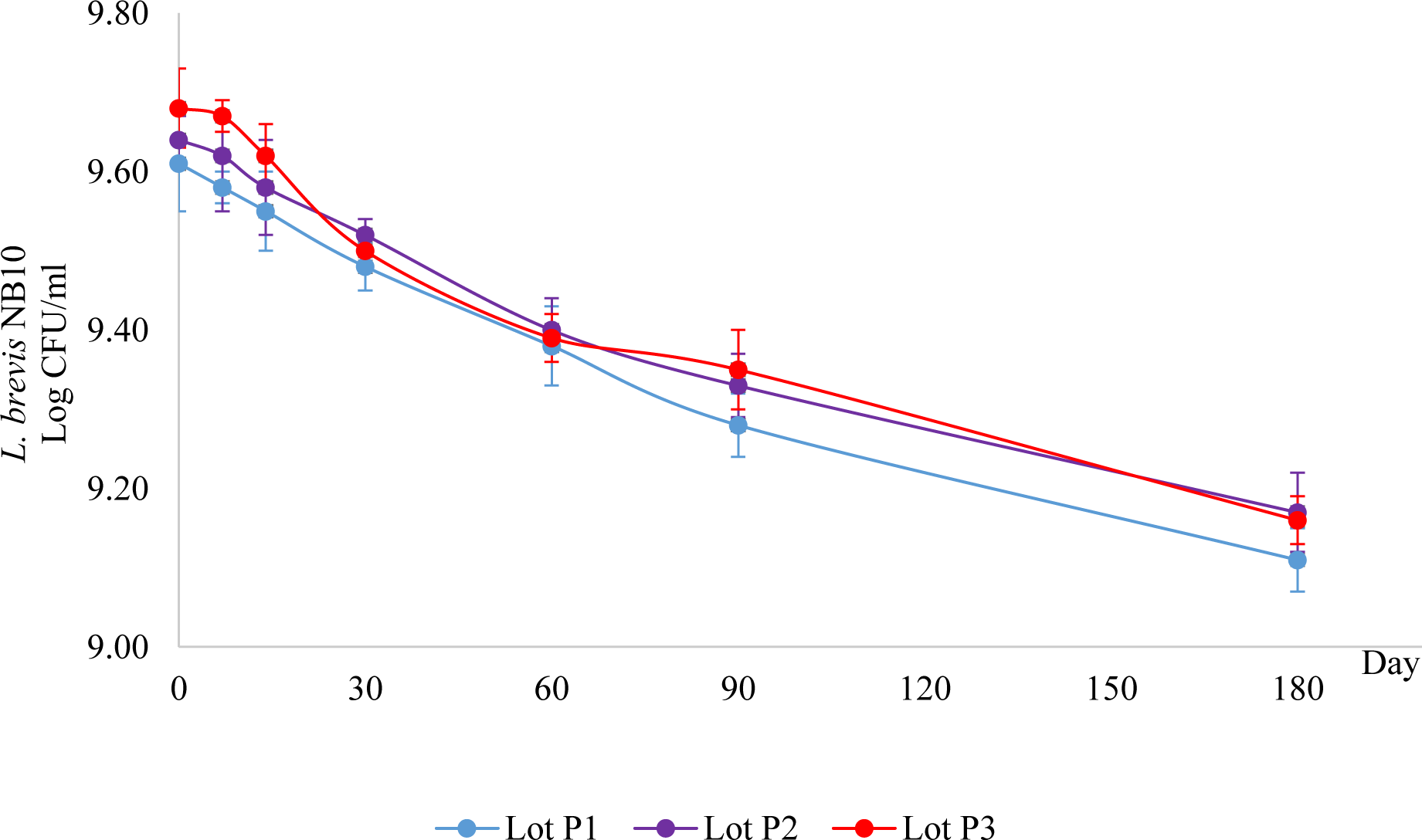
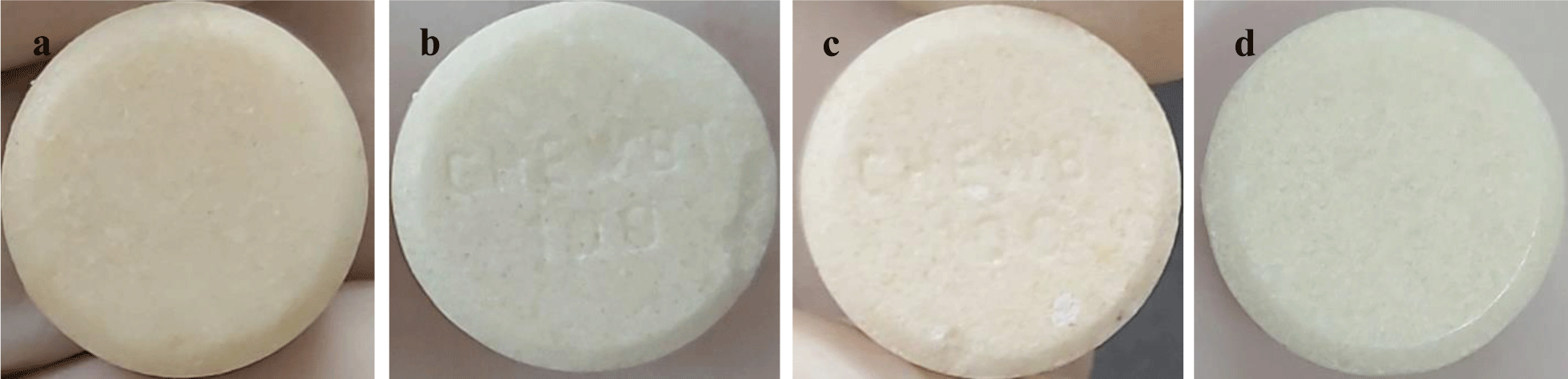
From the basic formulation, a series of formulations (F1 – F20) were made in which individual excipient was changed at a time to examine the effect of its on the product properties (Table 9). The formulations F1 – F4 were used to optimize chewable gum mass; F5 – F9 for selection of suitable sweetness; F10 – F13 for adjusting gum flavor intense and lasting time; F14 – F17 for modifying gum softness; and F18 – F20 for prevention of the adhesion of the gum tablet into die and punches. The number of gum tablets prepared from each formulation was 30.
To obtain optimal gum mass for chewing, four formulations (F1-F4) were made and evaluated by healthy human volunteers (n = 6). The number of viable L. brevis NB10 and chewing feel scores from response of human testers were shown in Table 10.
The average number of L. brevis per tablet in all formulations was higher than 107 CFU. The formulation F3 (1800 mg) was rated as the most suitable size for chewing. Hence, the total mass of gum tablets prepared for subsequent experiments was fixed as 1800 mg.
The freeze-dried L. brevis NB10 powder has unpleasant taste, thus addition of sweetener is necessary to achieve better taste and enhance attraction to consumers. Xylitol was selected as sweetener in this study because of its anticariogenic ability and safeness [18], [19], [20].
The increase of xylitol concentration in gum formulations (F5 – F9) enhanced the sweetness of the gum tablets while not affected the viability of L. brevis NB10 (Table 11). The formulation F7 showed the most appropiate sweetness (according to 5/6 testers). Hence, the concetration of xylitol used in gum formulation was selected as 10% (w/w) (180 mg/1800 mg of gum tablet).
Like sweeteners, flavors are important ingredients in gum formulation which provide product attractive scent. Spearmint and peppermint are among the most used flavor in chewing gum [21]. However, these mints contain essential oil components which can exert antibacterial effect, and hence, can affect the viability of L. brevis NB10 in the formulation. Thus, flavor from fruits, i.e., strawberry flavor powder in this study was chosen to adjust gum flavor.
From the results shown in table 10, strawberry flavor did not cause negative effects on L. brevis NB10 during formulation. The gum flavor become more intense and longer-lasting when increasing the concentration of strawberry flavor, wherein, the formulations F12 and F13 showed good lasting flavor (> 5 mins) (Table 12). However, the formulation F12 had the most acceptable flavor as voted by 6/6 tester. Hence, the concentration of strawberry flavor used in gum formulation was set as 2% (w/w) (36 mg/ 180 mg of gum tablet)
Formulation | ||||
---|---|---|---|---|
F10 | F11 | F12 | F13 | |
Flavor lasting time (second) | 61.5 ± 10.5 | 178.8 ± 10.3 | 329.5 ± 31.8 | 389 ± 16.9 |
Chewing gums should have the proper softness to confer a comfortable chewing feel. If the gum texture physically becomes too rigid or too soft, it will make the chewing feel less joy and reduce the consumer interest in the product. Glycerol is used widely in chewing gum formulation as moisture-retaining and flexibility enhancing [22]. Also, glycerol has no data for its cariogenic effect.
The gum tablet texture from the formulation F14 - F17 became softer when the glycerol concentration increased, wherein the gum tablet made from F16 showed the most appropriate softness for chewing (rated by 5/6 testers) (Table 11). Therefore, the concentration of glycerol used in the formulation was fixed as 1.5 % (w/w) (27 mg/ 180 mg of gum tablet).
Through a series of screening experiments for sweetener, flavor, and softener, the L. brevis NB10 chewing gum formulation consisted of 10% L. brevis NB10 freeze-dried powder, 10% xylitol, 2% strawberry flavor powder, 1.5% glycerol. Flow properties of the gum powder (before compression) from this formulation showed good flowability. The angle of repose was 33.66 ± 0.38 (≤ 35 for good flowability) and the Hausner ratio was 1.14 ± 0,00 (≤ 1.18 for good flowability). Also, the compressibility index of the gum powder was 12.57 ± 0.13 which is considered good compressibility and suitable for the direct compression method. However, gum tablets obtained from this formulation were shown to adhere to die and punches of tablet compression machine. Hence, lubricants such as talc, magnesium stearate were added to overcome this issue (F18 – F20). The properties of gum powders and gum tablets from these formulations were shown (Table 13).
The addition of talc and magnesium stearate into gum formulations did not cause harmful effects on L. brevis NB10 viability during gum preparation. Also, these lubricants did reduce the sticking of tablets to die and punches and made them easier to eject from the die. However, the use of 3% talc (w/w) in the formulation F18 may reduce the adhesion of the gum powder particles, which in turn, make the gum tablet surface become cracking after compression. Meanwhile, in the formulation F19, magnesium stearate at 3% (w/w) was not completely dispersed throughout the gum powder mixture, thus, the gum tablet surface from F19 showed some white spots and lack of uniformity. In the formulation F20, when adding 3% (w/w) of talc: magnesium stearate mixture (1:1), the gum tablets were perfectly made without cracking and flaw on the surface. Therefore, formulation F20 was chosen as the final formulation of L. brevis NB10 chewing gum.
To evaluate the consistence of gum tablet formulation and compression process, 3 lots of L. brevis NB10 chewing gum were made. The number of tablets made for each lot was 100 tablets. The number of viable L. brevis NB10 in gum powders and tablets from each lot showed no significant difference (Table 14).
Next, the bacterial stability in gum tablets from these three lots at 5 °C ± 3 °C were studied. The average numbers of live L. brevis NB10 in the gum tablets in 90 days of storage were shown below (Figure 5).
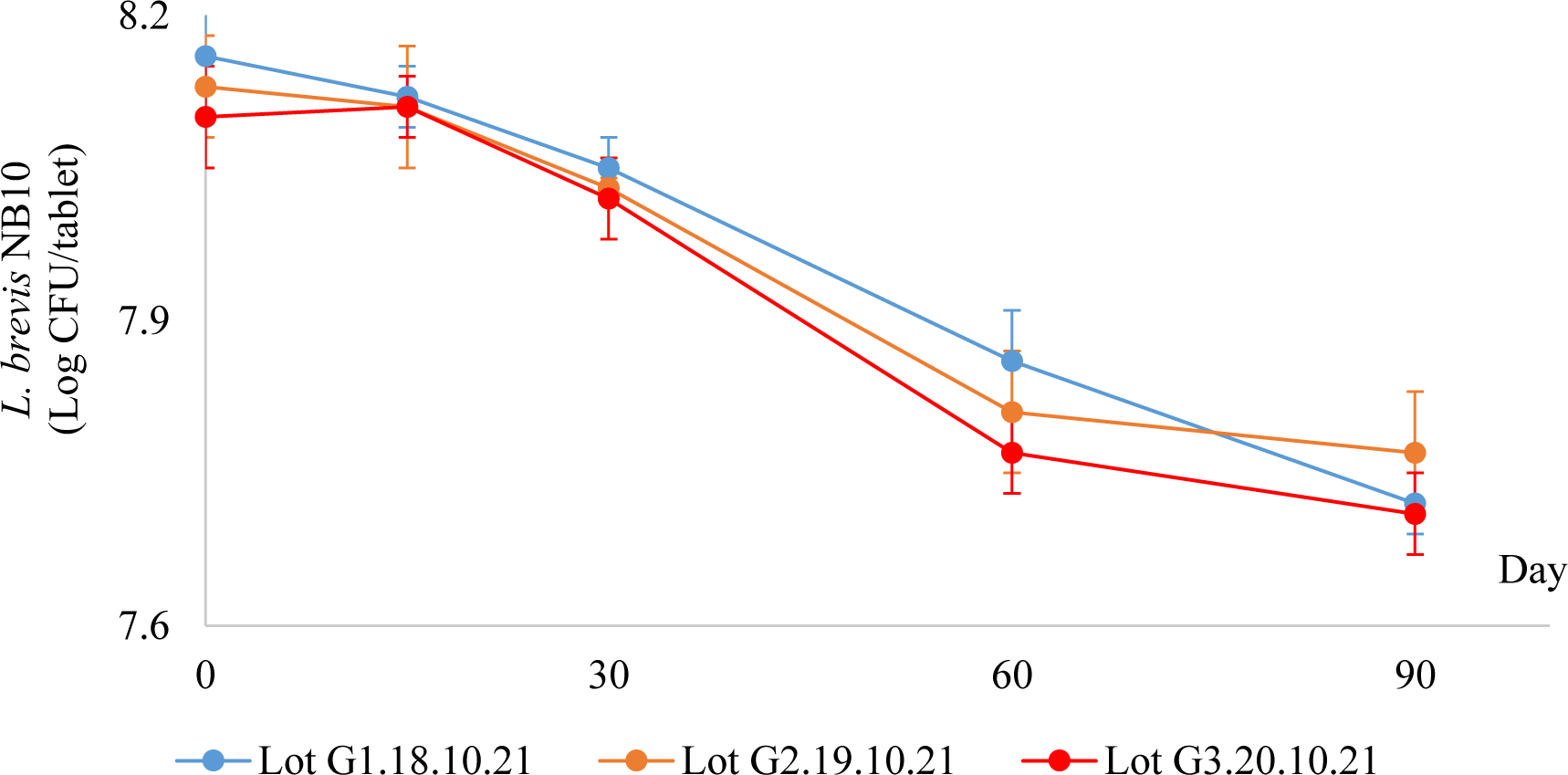
In all chewing gum lots, the number of live L. brevis NB10 in gum tablets was decreased over time but remained higher than 107 CFU/tablet in 3 months of storage. Therefore, the chewing gum product was shown to be stable at 5 °C ± 3 °C for at least 3 months.
4. DISCUSSION
Freeze-drying (as known as lyophilization) is used widely as a preservative method for various kinds of pharmaceutical and biological products such as antibiotics, vaccines, recombinant proteins, biologics, and probiotics. Freeze-drying takes place in 3 stages: (1) freezing – the product is frozen at ultra-low temperate so as all the water (or solvent) in the formulation becomes ice (crystallization); (2) primary drying – most of the water content in the product as ice crystals is removed through sublimation process at low pressure; (3) secondary drying – the remaining water is further removed from the product by desorption [23]. During the freeze-drying process, microorganisms are suffered from different stresses such as cold shock, osmotic stress (due to the concentration of solutes), mechanical forces such as lethal damage from ice crystals. Also, the storage and the rehydration conditions of the products also strongly affect the viability of bacteria [23 - 25].
Methods used to improve bacterial survival rate in the freeze-dried product include (1) adjustment of culture condition and pre-stressing strategies to alter the composition of bacterial cell wall and membrane which help to increase the tolerance of bacteria to cold and water loss effect; (2) formulation of suitable protective media which help to reduce the negative effect of mechanical and osmotic stresses during freezing stage (cryoprotectants) or during drying stages (lyoprotectants); (3) optimization of freeze-drying process parameters such as freezing temperature and cooling rate (which results in different size and amount of ice crystal) or the temperate and pressure at which the drying stages take place (which affect the time needed to complete the process). While the screening of culture conditions is time-consuming and cannot replace the need for the two latter strategies, the optimization of lyophilization parameters requires sophisticated equipment and depends on the protective formulation used. Optimization of the freeze-drying formulation is often the method of choice [23 - 25].
Various types of substances were studied as protective agents for preserving probiotics during the freeze-drying process. However, their mode of action was not yet fully understood. Low molecular sugars (glucose, lactose, trehalose…), polyols (sorbitol, mannitol, xylitol…), or amino acid (lysine, glycine, glutamine…) can act as water substitutions to bind to bacterial phospholipids and proteins, thus, reducing the damage to these structures during freezing, dehydration, or rehydration process. Large molecular protectants such as polysaccharides (maltodextrin, inulin, FOS, GOS…) or skim milk tend to accumulate outside of the cells and enlarge the unfrozen fractions during the freezing stage, thus, reducing the harmful effects of mechanical and osmotic stresses. Also, most protective agents can lower the nucleation temperature. Therefore, they can reduce the size of ice crystals and their damage to the cells. However, there is no such universal formulation of protectants that is suitable for all bacteria. The effects of protective agents are different for each strain [24, 25].
To prepare a freeze-dried powder of L. brevis NB10 for use in chewing gum, protective agents that exhibit low or no cariogenic activities were selected [25]. As such, mannitol, xylitol, sorbitol, lactose, maltodextrin, and skim milk were screened to design the freeze-dried formulation that provided the highest L. brevis NB10 viability and stability. The results showed that the combination of protectants showed a better protective effect on L. brevis NB10 than each protectant when used alone, wherein, the mixture of maltodextrin 6% and skim milk 6% showing the best performance. The augmented effect when using mixture of protectants was also observed from different studies (Table 15). Also, the suitable protectants and their use amount are varied for each strain.
Lactobacilli | Optimal freeze-drying formulation | % survival | Ref |
---|---|---|---|
L. acidophilus (NCDC 291) | 1.2% Sucrose + 6% skim milk | 69.86 | [27] |
L. acidophilus (NCDC 015) | 68.84 | ||
L. acidophilus (MTCC 10307) | 67.92 | ||
L. lactis Sr. 3.54 | 10% (Sucrose + skim milk) | 62.0 | [28] |
L. paracasei subsp. tolerance (DSM 20258) | 6% Skim milk + 8% Trehalose + 4% sodium ascorbate | 82.0 | [29] |
L. delbrueckii subsp. bulgaricus (DSM 20081) | 74.0 | ||
L. plantarum JH287 | 10% Skim milk + 10% Sucrose | 77.15 | [30] |
L. brevis NB10 | 6% maltodextrin + 6% skim milk + 0.25% potassium ascorbate | 75.5 | This study |
As a probiotic containing chewing gum, the exposure of freeze-fried bacteria to water, solvent, high temperature, oxygen… during the preparation process affect greatly on its survival in the product tablets. Thus, the direct compression method using gum base was chosen to reduce the preparation time and get rid of harmful events. Other excipients were examined one by one for the selection of suitable amounts and suspected of any negative effect on L. brevis NB10 viability.
Though not showing the protective effect on L. brevis NB10 during the freeze-drying process in this study, xylitol is a common sweetener in chewing gum formulation. Due to its anti-cariogenic effect, the combination of xylitol and L. brevis NB10 may show the synergic effect on the prevention of tooth decay caused by Streptococcus mutans.
In this study, healthy human volunteers (n = 6) were recruited to evaluate some organoleptic properties of chewing gum tablets such as chewability mass, sweetness, flavor lasting time, and softness to optimize the amount of each excipient. All chewing gum tablets used in human evaluation tests were prepared using placebo freeze-dried powder which was not contained live L. brevis NB10. The limitation of the current study was the low number of human participants. Therefore, the organoleptic evaluation recorded by this study might not represent the feedback of larger group of people. However, all the excipients used in the chewing gum formulation did not affect the viability of L. brevis NB10 during the preparation process. Thus, if using a larger number of human testers to further optimize chewing gum formulation, one can easily re-establish the amount of each excipient without affecting the survival rate of L. brevis NB10 as achieved in this study.
Conclusion
This study was successful in formulating chewing gum containing at least 107 CFU/tablet of L. brevis NB10. The prepared chewing gums were stable for at least 3 months at 5 °C ± 3 °C. Further studies could be conducted to improve bacterial stability such as anti-moisture film coating or optimization of the preparation process.