1. INTRODUCTION
A beautiful smile instills confidence in humans’ daily social interactions. Smile aesthetics are assessed not only based on tooth ap-pearance but also influenced by factors related to the gingiva tissue, such as gumline contours, gingival color, the harmonious balance of gingiva alongside the teeth, etc. Gingival recession is a significant factor that diminishes the beauty of a smile. In addition to exter-nal gum recession, there are functional consequences related to tooth protection. The gum recession between teeth is also receiving significant attention due to its impact on aesthetics and functionality. According to Morris et al. (2017), the percentage of patients with gum recession after orthodontic treatment is 18.3% [1].
Many recent studies have focused on the application of biocompatible substances in tissue regeneration therapies, including hyalu-ronic acid (HA). Author Becker et al. (2010) were the first to observe that minor gingival recessions could be regenerated using HA gel [2]. However, a study by Bertl et al. (2016) found that HA gel did not improve gingival recession in cases involving implant-supported restorations [3]. These findings highlight the necessity for further research on HA injection methods. In particular, in vitro studies are essential to better understanding this compound’s effects on gum tissue.
HA (C14H21NO11)n is a natural polysaccharide compound belonging to the non-sulfated glycosaminoglycans (GAGs). The HA poly-mer chain can have a repetition of as many as 25,000 units, and its molecular weight can vary between 5 and 20,000 kilodaltons (kDa) [4]. HA is one of the main components of the extracellular matrix in most human body cells. HA is most concentrated in con-nective tissues and least concentrated in the serum. In oral tissues, HA is found in non-mineralized tissues, including the gingiva and periodontal ligament, and in smaller amounts in mineralized tissues, such as cementum and the alveolar bone. In addition, HA is present in the gingival crevicular fluid and the non-stimulated saliva [5,6].
HA, in its various concentrations and molecular weights, elicits different cellular resposnses. High molecular weight HA (>5,000 kDa) inhibits angiogenesis and promote immune suppression. Conversely, medium molecular weight HA (20–1,000 kDa) plays a vital role in biological processes, such as wound healing and regeneration. Low molecular weight HA (6–20 kDa) contributes to the cellular processes, including inflammation, angiogenesis, and gene expression [6]. Several studies have highlighted its anti-inflammatory prop-erties, water retention capabilities, and antimicrobial properties. HA has anti-inflammatory effects by reducing the formation of matrix metalloproteinase (MMP), preventing the synthesis of prostaglandin E2 (PGE2) and bradykinin, inhibiting the formation and activity of interleukin (IL) 1β, and other pro-inflammatory mediators [4,7–9]. In an in vitro investigation, Sasaki proposed that the inflammatory upregulation of MMP-1 and MMP-3 triggered by IL-1β in human synovial cells is carefully controlled by HA. HA can impede the interaction of IL-1β with its cell membrane receptor by enveloping the cell surface, consequently hindering the production of MMPs. This suggests HA injections could reduce tissue destruction in inflamed joints, including bone and cartilage degradation. Additionally, Yasui also reported the ability of HA to inhibit the production of PGE2 by IL-1α in human synovial fibroblasts [10,11].
A notable property of HA is its hydrophilic nature and its ability to undergo hydration to form a gel-like structure. It is one of the most moisture-absorbing molecules known in nature. When HA interacts with water molecules, hydroxyl bonds are formed between the adjacent carboxyl and N-acetyl groups, allowing HA to retain water and maintain a sturdy structure. These biological properties regu-late osmotic pressure, adjusting the viscosity of biological fluids, preserving structural integrity and stability, and maintaining internal equilibrium [12]. The antibacterial property of HA has been demonstrated through clinical efficacy in treating inflammatory and infec-tious diseases. At high concentrations, medium and low molecular weight HA exhibits antimicrobial activity, particularly against Ag-gregatibacter actinomycetemcomitans, associated with periodontal inflammation. Furthermore, HA acts as a biological signal mole-cule for processes such as leukocyte proliferation, phagocytosis, cell growth, and migration, including fibroblasts. Due to these biologi-cal properties, HA helps reduce the invasion of bacteria and viruses into the body tissues [13].
Various factors influence the healing of gingiva in periodontal regeneration treatments. Fibroblasts, the predominant cell type in the cellular gingival tissue, play a crucial role in synthesizing and maintaining extracellular matrix components like collagen fibers, elastin fibers, glycoproteins, and GAGs. Upon tissue damage, fibroblasts migrate to the injured site, proliferate, and produce a substantial amount of extracellular matrix to aid in tissue repair [14].
To further our understanding of HA’s potential to support gingival healing, especially in periodontal regeneration treatments, we conducted an in vitro study to assess its effect on the proliferation and migration of human gingival fibroblasts (hGFs).
2. MATERIALS AND METHODS
The study utilized a commercially available HA product, Bioregen® Regenyal, imported from Regenyal Laboratories S.R.L, Italy, by the Vietnam Ministry of Health under import license number 10058NK/BYT-TB-CT. This product contained HA at a concentra-tion of 16 mg/mL, derived from microbial fermentation. The composition included 1 mL of sodium chloride, phosphate buffer, and distilled water. The HA underwent a fragmentation process initiated from one megadalton (MDa) fraction. This fragmentation process incolved the application of heat and ultrasonic waves in a strongly alkaline environment. resulting in distinct molecular fractions with various molecular weights (2, 100, 200, 500 kDa, and 1 MDa), each tailored for specific functions.
In this study, hGFs at passage 3, which were available from the cellular repository of the Laboratory of Tissue Engineering and Bi-omedical Materials, Department of Physiology and Animal Biotechnology, Faculty of Biology—Biotechnology, University of Science, Ho Chi Minh City, were used. Gingival tissue was discarded from healthy patients who underwent gingivectomy in the maxillary ante-rior region for aesthetic purposes. Culture, isolation, and characterization of hGFs were conducted following the laboratory’s procedure. After two weeks of incubation, when human gingival hGFs achieved 80%–90% confluence, they were assessed for expression of cellu-lar markets. The cells exhibited positive staining for mesenchymal cell marker Vimentin and negative staining for epithelial cell marker Cytokeratin [15–17], confirming their mesenchymal origin. Cells were cultured up to passage 4 using a growth medium consisting of Dulbecco’s modified Eagle Medium: nutrient mixture F-12 (DMEM/F12) supplemented with fetal bovine serum (10%FBS), streptomy-cin (100 µg/mL), and penicillin (100 IU/mL) (Sigma-Aldrich, St. Louis, MO, USA). Under these conditions, hGFs display adherence to and exhibited the characteristic spindle shape type of fibroblasts. In the incubator, hGFs were maintained at 37℃, 5% CO2, and ob-tained the confluence of 80%. For this study, we used cells from passages 4–6 (Fig. 1).
The study was approved by the Ethics Board of the University of Medicine and Pharmacy at Ho Chi Minh City (No. 665/HDDD-DHYD).
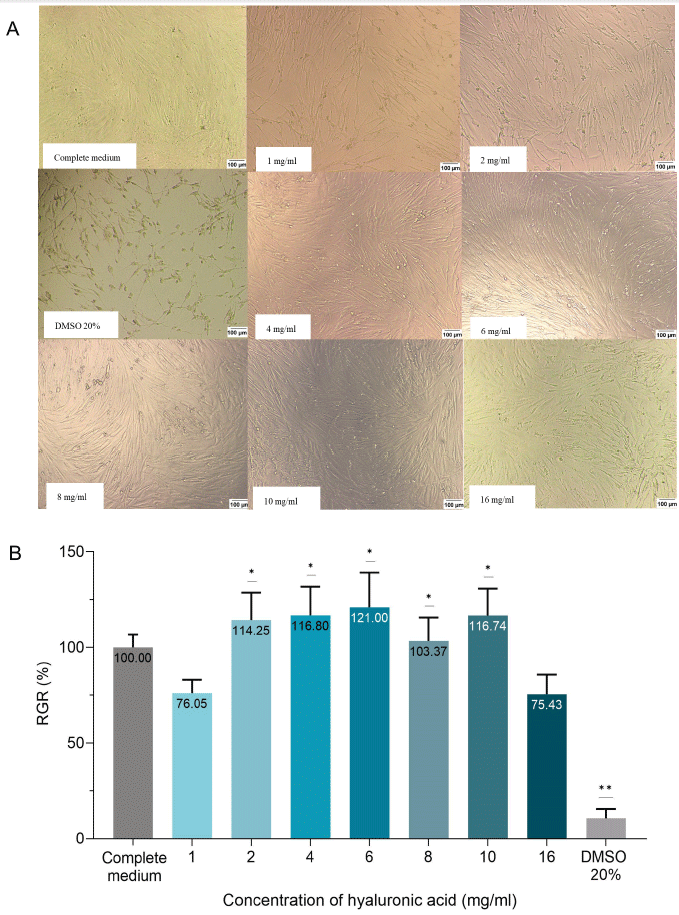
The influence of HA on cell viability was evaluated by the colorimetric tetrazolium salt assay, known as [3-(4,5-dimethylthiazol-2-yl)-2,5-diphenyl tetrazolium bromide] (MTT) assay. 104 cells/well of hGFs were seeded in 96-well microplates. After 24 hours, HA at various concentrations of 1, 2, 4, 6, 8, 10, and 16 mg/mL (diluted in serum-free medium DMEM/F12) or complete medium (CM) was added in triplicate to the 96-well/plates. The negative control group was the dimethyl sulfoxide (DMSO) 20% solution, which has been proven to induce cell death [18]. After 24 hours, the cell culture was examined microscopically for cellular morphology. MTT solution was added to the plates after the medium was replaced. The incubation will last 4 hours at 37℃ to encourage the formation of forma-zan crystals. DMSO/Ethanol solution (Sigma-Aldrich) was used to dissolve crystals with the creation of the purple solution. A micro-plate photometer (Biochrom, Cambridge, UK) was used to measure the optical densities (OD) at a wavelength of 570 nm. According to ISO 10993-5, the relative growth rate (RGR) was calculated by the formula: %RGR = [OD of the HA treated group / OD of the CM group] × 100%. If the RGR value is over 70%, the HA is considered non-cytotoxic to hGFs [19].
The impact of HA on hGF proliferation was assessed using the hemocytometer cell counting method. A total of 103 cells/well of hGFs were seeded into 96-well microplates and cultured in HA at non-cytotoxic concentrations diluted in serum-free medium (DMEM/F12) for 24 hours. The positive control group received CM (10%FBS/DMEM/F12) while the negative control group was treat-ed with serum-free medium (S-fM, DMEM/F12). Each experimental and control group was replicated three times, with medium re-placement every two days. Cell numbers were measured at the intervals of 1, 3, 5, 7, and 9 days. To detach the cells, 0.25% trypsin / ethylenediaminetetraacetic acid (EDTA) was used, followed by staining with 0.4% trypan blue, then loaded onto a hemocytometer and using a light microscope (CKX53, Olympus, Tokyo, Japan) to count the cell number.
The impact of HA on hGF migration was observed in the scratch wound healing assay. In detail, 105 cells/well of hGFs were seeded in a 6-well plate and cultured to 80% confluence. Following 24 hours of starvation, a linear wound was created on the culture surface in each well using a sterile 100 μL-pipette tip. Non-adherent cells were removed by washing with phosphate-buffered saline (PBS). HA at non-cytotoxic concentrations, diluted in DMEM/F12, was added in triplicate to the 6-well plate, and cells were cultured for an addi-tional 24 hours. At the time points of 0, 18, and 24 h, the migration of hGFs to the empty scratch area was observed with a phase-contrast microscope (CKX53, Olympus) and recorded by imaging software (cellSens, Olympus). The images of cell-free areas were analyzed using Fiji: ImageJ 1.53 software and the “wound healing size tool” as the previous study [20]. Positive (CM, 10%FBS/DMEM/F12) and negative (S-fM, DMEM/F12) control groups were included for comparison.
The data are presented as means±SD. Statistical significance was determined using T-test for paired groups, while one-way ANOVA multiple comparisons with Bonferroni correction was used for comparisons between multiple groups. GraphPad Prism, version 9.5 (GraphPad Software, San Diego, CA, USA) was utilized for statistical analysis. A p-value below 0.05 was considered statistically sig-nificant.
3. RESULTS
After 24 hours of incubation with cell culture wells containing various concentrations (1, 2, 4, 6, 8, 10, 16 mg/mL) of HA, hGFs ad-hered and maintained their characteristic spindle shape, which was consistent with the morphology of untreated cell in CM (10%FBS/DMEM/F12). Subsequent incubation in the MTT solution resulted in all seven HA groups at different mentioned concentra-tions exhibiting a purple coloration indicative of formazan crystals covering almost all the well surfaces, similar to that in the CM group.
The results of the RGR in the experimental groups have been illustrated in Fig. 2. In particular, the RGR in the 1, 2, 4, 6, 8, 10, and 16 mg/mL HA groups was 76.05%, 114.25%, 116.80%, 121.00%, 103.37%, 116.74%, and 75.43%, respectively, which was upper 70%, indicating the limit of non-cytotoxic determination of the test sample. Significant differences existed between the RGR value in the 2, 4, 6, 8, and 10 mg/mL HA groups and the value of 70% (One sample t-test). According to ISO 10993-5 standard, HA at the concentrations (from 1 to 16 mg/mL) with percentages of viable cells higher than 70% after 24 hours were non-cytotoxic to hGFs. There was no significant difference between the value of RGR in the 1, 2, 4, 6, 8, 10, and 16 mg/mL HA group (One-way ANOVA test).
In the cell viability assay, HA concentrations of 2 and 6 mg/mL (diluted in DMEM/F12) were identified as non-cytotoxic and se-lected as experimental groups to assess their impact on hGF proliferation.
In the CM group, hGFs showed a notable proliferation from the first to the third day, reaching a peak on the 5th day, followed by a gradual decline on the 7th and 9th day. Statistical analysis revealed that the cell proliferation on the 5th day was significantly higher compared to that of the first day (p<0.0001) (Fig. 2).
In the 2 mg/mL HA hGFs exhibited an increase in cell number on the 3rd day, reaching a peak on the 5th day. The difference be-tween the cell count on the first and the 5th day was statistically significant (p=0.0014) (Fig. 2).
In the 6 mg/mL HA group (Fig. 3), the proliferation rate of hGF was nearly similar to that in the CM group. The highest number of hGFs on day 5 was statistically superior to that of the first day (p=0.0074) (Fig. 2).
When compared to the S-fM group, the hGF number in the HA 2 mg/mL group was significantly higher on day 3 (p=0.0051). Nota-bly, the proliferation of hGFs in the HA 6 mg/mL group exhibited statistically cell numbers compared to the S-fM group on days 1, 3, 5, 7, and 9 (Fig. 2).
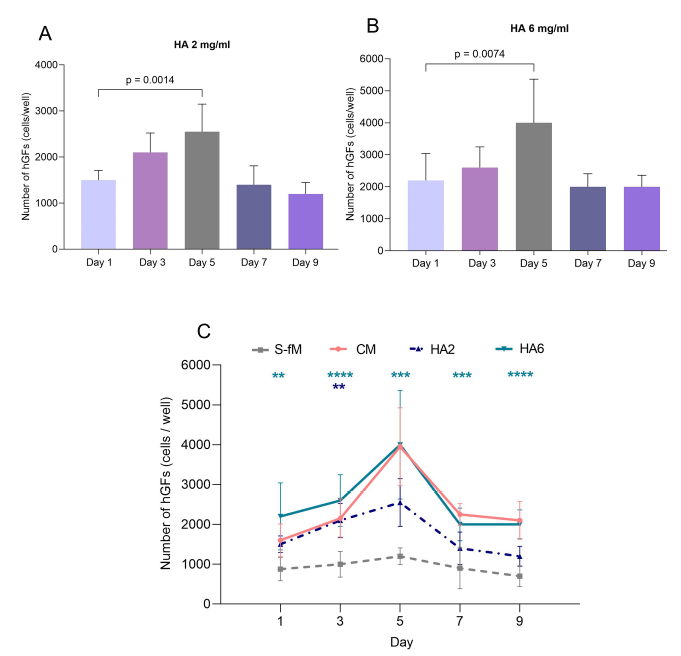
The ability of hGF migration affected by HA was observed in the scratch-test assay and presented as a percent change in the area between the dashed lines. The scratch area at 0 hours was standardized as 100% (Fig. 3). Similarities existed between the HA 2 mg/mL and the S-fM group, where the percentage of cell-free areas was statistically unchanged after 18 and 24 hours. The remaining empty cell area in the CM group gradually decreased after 18 hours and 24 hours, compared to the time point of 0 hours. Moreover, in the mentioned group, the statistical analysis showed that the gap area was also narrowed between 18 and 24 hours. In the HA 6 mg/mL group, the wound closure after 18 and 24 hours had significant differences compared to 0 hours. However, a significant difference was not statistically recorded between these two time points. Interestingly, the migration of cells in the HA 6 mg/mL group was indicated as the strongest, where the remaining wound area percentage at 18 hours and 24 hours was 55.73% and 51.32%, respectively. The results showed that HA 6 mg/mL significantly induced fibroblast cell migration compared to the S-fM group (p<0.0001) (Fig. 3).
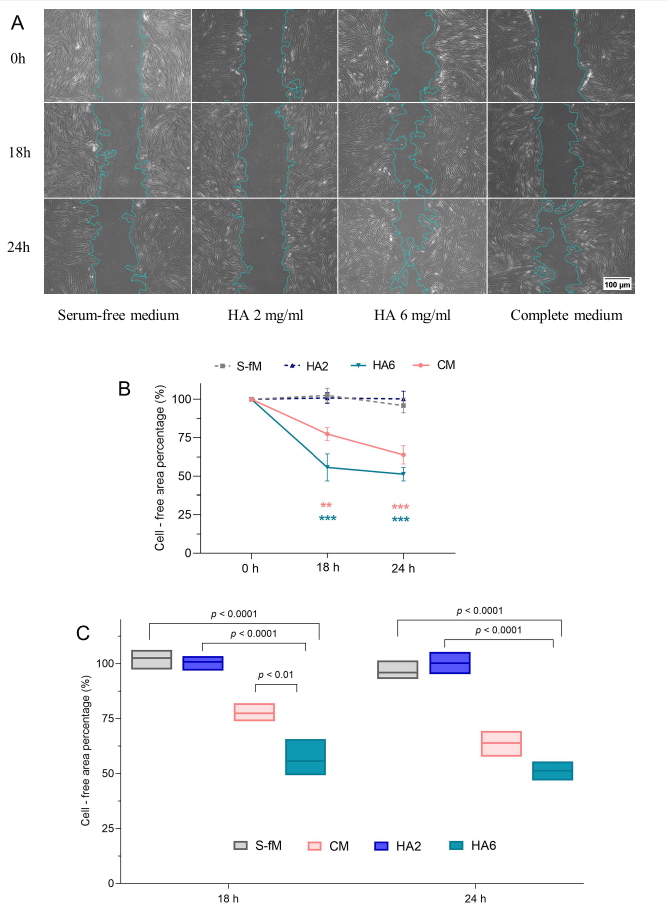
4. DISCUSSION
hGFs, constituting a significant element within the gingival connective tissue, assume a pivotal function during the healing process in gingival wounds. Moreover, hGFs have a high proliferation capacity in vitro, even under less strict culture conditions, making them a valuable source for basic research [21]. Studying hGFs provides insight into the mechanisms and evidence for oral wound healing. hGFs used in the study were isolated and cultured following the method outlined by author Nguyen et al. in 2009 [16]. The cells were cryopreserved until needed, thawed, and subcultured to the third passage (P3). Upon observing the morphology of gingival fibroblasts, we noted their consistent characteristic shape of elongated spindle or star-like shapes. They spread across the culture dish surface and formed spiral patterns. At a cell density of 70%–80%, cells were sub-cultured to passage 4 (P4) to increase cell numbers for research purposes while ensuring nutritional factors and space for cell growth. hGFs maintained their biological characteristics and proliferative potential across multiple passages. In this study, we chose hGFs at P4 to P6 to ensure consistent morphology and biological features, reducing test discrepancies. Some studies also employed cells from P3 to P6 for similar purposes [22,23].
Regarding this study, an MTT assay was utilized to assess the cytotoxicity of HA to hGFs. The MTT assay converts MTT into pur-ple crystals in viable cells, which are then solubilized in DMSO/Ethanol. This indirect approach is reflected in the RGR because the optical density values of the formazan solution correlate with the number of viable cells. According to ISO 10993-5 standards, if via-ble cells are <70%, the test sample is considered cytotoxic [19]. In our study, the MTT results after 24 hours demonstrated that HA at concentrations of 1 to 16 mg/mL did not influence the viability of hGFs. This finding resembles the results of Asparuhova et al. (2018), who assessed the toxicity of HA gel on hGFs and observed that concentrations of HA in the range of 0–4 mg/mL did not cause cyto-toxicity [24]. Additionally, our study evaluated higher concentrations such as 6, 8, and 10 mg/mL, which also did not affect the via-bility of hGFs, with cell viability ratios in HA groups exceeding 100%.
In terms of the proliferation of hGFs cultured in various types of medium, including CM, serum-free medium (S-fM), 2 mg/mL of HA, and 6 mg/mL of HA, the cell count follows the typical growth curve of cells: lag phase, log phase, stationary phase, and death phase. HGFs grown in the CM group were supplemented with 10% FBS, which contains growth factors, minerals, lipids, proteins, and hormones necessary for cell survival and promoting cell development and adhesion. It gradually increased from the first to the third day, peaked on the 5th day, and decreased from the 5th to the 9th day. Regarding the S-fM group without FBS, the number of hGFs was lower than that of the positive control group at all time points: days 1, 3, 5, 7, and 9. Our study used DMEM/F12 medium without serum to dilute HA to evaluate its effect on the proliferative capacity of hGFs. In the HA group with a 6 mg/mL concentration, hGFs showed statistically significant proliferation from day 1 to day 5 compared to the negative control and positive control groups. This finding is consistent with the Ni et al. (2021) report, which observed significant fibroblast proliferation in HA at the concentration of 6% compared to the control group from day 2 to day 4 [25].
The mechanism of migration assay employed chemotaxis, where injured cells emit signals, causing remaining cells to migrate to-wards areas with increased signal concentration, aiding wound healing and tissue formation. The cell-free percentage area indicated the number of migrated cells. Based on the results, at the 18-hour, hGFs in the HA 6 mg/mL group exhibited significantly more migra-tion than the negative control and positive control groups, which was statistically significant. This result aligns with the findings of Asparuhova et al. (2018), where at the 18-hour time point, HA at the concentration of 4 mg/mL positively influenced the migration ability of HGF cells compared to the control group [24]. Our study found that hGFs in the HA group at 6 mg/mL showed significantly more migration than in the negative control group at the 24-hour mark, within the fibroblast proliferation cycle from 24 hours [26]. The scratch test resulted in observed wound healing within 24 hours due to cell migration, independent of cell proliferation.
Here are some explanations of how HA can affect cell proliferation and migration: in numerous reports, the cellular signaling and activation of receptors by HA contribute to the activation of growth factors. HA interacts with cells through receptors, mainly with CD44 (Cluster of Differentiation 44) and RHAMM receptors (Receptor for Hyaluronan-Mediated Motility). The interactions with these receptors trigger signaling pathways that regulate cell functions, including proliferation and migration. CD44 is the primary receptor for HA, found on the cell membrane of most human cells. Hirano et al. reported the CD44 receptors and mRNA expression in human fibroblasts derived from gingival and periodontal ligament and gingival epithelial cells. CD44 and HA are not capable of independent action [27].
In wound sites, CD44 is responsible for the internalization of degraded HA products that transmit cell signals, and it plays a critical role in the inflammatory response. CD44 also helps attract hGFs from surrounding tissues to the wound area, activating signals that support cell migration and development [28]. Additionally, HA facilitates the proliferation of hGFs through the transforming growth factor - β1 (TGF-β1) pathway via interaction between CD44 and epidermal growth factor receptor (EGFR), subsequently promoting the specialized mitogen-activated protein kinase (MAPK)/ extracellular signal-regulated kinase (ERK) pathway, leading to robust cell pro-liferation [29]. Studies by Ohno and Asparuhova demonstrated that HA activates ERK1, phosphoinositide 3-kinase (PI3K) / protein kinase B (AKT), and p38 MAPK signals, enhancing cell viability, proliferation, and migration abilities [24,30].
5. CONCLUSION
Within the limits of the in vitro study, the results showed that HA was a non-cytotoxic active compound that supports the prolifera-tion and migration of gingival fibroblasts. The study lays the foundation for applying HA in clinical periodontal regeneration, particu-larly interdental papilla loss treatment. To better understand the direct clinical use of HA, we will conduct further research on im-provements in HA, such as ingredients and additives.