1. INTRODUCTION
Acute respiratory distress syndrome (ARDS) is a pulmonary inflammatory condition resulting in non-hydrostatic protein-rich pulmonary edema [1]. Positive end-expiratory pressure (PEEP) counterbalances these adverse forces by promoting lung recruitment and reducing mechanical heterogeneity [2]. Achieving “the best PEEP” is impossible. Instead, selecting a “better PEEP” guided by transpulmonary end-expiratory pressure (PL-exp) is a more appropriate approach. Maintaining PEEP to keep a positive transpulmonary pressure reduces atelectasis as well as cyclical opening and closing of alveoli to improve pulmonary mechanics and adequate oxygenation [3]. The use of esophageal pressure manometry to calculate transpulmonary pressure for optimizing and individualizing ventilator settings in patients with suspected high pleural pressures and refractory hypoxemia has been recommended [4,5]. However, the clinical implementation of esophageal pressure manometry placement remains limited. Thus, our study aims to observe the effect of oxygenation index and respiratory mechanics at 48h when PEEP is adjusted based on transpulmonary pressure.
2. MATERIALS AND METHODS
We collected data on all patients over 16 years of age who were diagnosed with moderate to severe ARDS by the Berlin Definition [6] criteria and admitted to the mixed intensive care unit (ICU) of a tertiary hospital, Cho Ray Hospital in Vietnam from November 2021 to October 2023.
Exclusion criteria included patients who underwent extracorporeal membrane oxygenation, prone-position ventilation, contraindications for esophageal pressure catheter placement, severe coagulopathy, pulmonary embolism, lung transplant, active bronchopleural fistula, neuromuscular disease, history of chronic obstructive pulmonary disease, absence of appropriate tools, or who declined to participate in the study.
All patients who met ARDS criteria and received the mechanical ventilation approach that followed the ARDSNet study to parameters aimed at maximizing respiratory function [7]. The ventilation was initiated with low tidal volumes of 6 to 8 mL/kg of predicted body weight (PBW) and the inspiratory plateau pressure was maintained below 30 cmH2O. If the plateau pressures was above 30 cmH2O at a tidal volume (VT) of 6 mL/kg PBW, it is acceptable to gradually decrease the VT to 4 mL/kg PBW. This adjustment includes setting a new limit for the plateau pressure at 35 cmH2O. The optimal level of oxygenation was closely monitored to arterial oxygen saturation within a range of 88%–95% or partial pressure of arterial oxygen (PaO2) between 55–80 mmHg. The respiration rate was adjusted to not exceed 35 breaths per minute, aiming for an arterial pH between 7.30 and 7.45. The PEEP was modified to improve oxygenation while minimizing the use of high fractions of inspired oxygen (FiO2) to prevent any negative effects on hemodynamic function, as shown in Table 1.
Following the initial ventilator setting, an esophageal pressure balloon (Nutrivent™, Mirandola, Italy) was placed in the patients. Begin by inflating the balloon with 6 mL of air to ensure its integrity and check for leaks, apply gentle pressure with the fingers to test tightness. After this inspection, deflate the balloon, connect the catheter to a three-way stopcock, and calculate the length of the catheter necessary from the xiphoid process to the earlobe and tip of the nose. Observe the esophageal pressure waveform on the ventilator to confirm a reading of 0±0.5 cmH2O, which ensures accuracy. Next, lubricate the catheter tip and insert the catheter transnasally or transorally until the balloon is 50–60 cm into the stomach. The position of the balloon is determined based on a chest X-ray at the bedside with a radiopaque marker on the balloon of the catheter. Fully deflate the balloon to equalize pressure before connecting it to the ventilator. Inflate the balloon gradually, following the principle of incremental volume from 0.5–8 mL. Measure the esophageal pressure corresponding to each volume, and plot the end-expiration and end-inspiration pressure-volume curve to determine the optimal balloon inflation volume whithin the linear segment. The catheter is retracted until cardiac oscillations appear on the waveform, indicating that the balloon has moved to the chest area. Finally, perform a dynamic occlusion test by compressing the chest during an expiratory hold and observe the variations in the ratio of esophageal pressure to airway pressure changes. A properly positioned catheter will exhibit a 1:1 ratio in pressure changes, with acceptable deviations between 0.8 to 1.2 in the transpulmonary pressure waveform.
During the observation period, a continuous infusion of sedatives and analgesics was administered to the patient. Additionally, rocuronium was provided as necessary for muscle relaxation. The “optimal” PEEP was established by achieving a minimal end-expiratory transpulmonary pressure range of 0–10 cmH2O, and arterial blood gases were examined. The measurement of intrinsic PEEP before and after each PEEP adjustment was conducted to ensure that the inspiratory/expiratory ratio was adjusted properly to prevent auto-PEEP.
Patient monitoring included age, sex, body mass index (BMI), risk factors for ARDS, Sequential Organ Failure Assessment (SOFA) score, and Acute Physiology and Chronic Health Evaluation II (APACHE II) score at ICU admission. All patients were monitored for arterial blood gas parameters, respiratory measures including transpulmonary end-expiratory/inspiratory pressure (PL-exp/insp), esophageal end-expiratory/inspiratory pressure (Pes-exp/insp), and hemodynamics at baseline, 24, and 48 hours. Primary outcomes were the PaO2/FiO2 ratio and respiratory mechanics parameters including airway driving pressure (DP), plateau pressure (Pplat), and respiratory system compliance (Crs). Secondary outcomes included hemodynamic parameters at 48 hours, the number of ventilator days, and length of ICU stay.
Transpulmonary pressure (PL) absolute values were calculated by airway pressure minus esophageal pressures (Pes):
Continuous variables were described as median and interquartile range (IQR), while categorical variables were described as frequency count and percentage. We analyzed differences in baseline, 24, and 48-hour measurements using ANOVA for Repeated Measures, and overall differences across time points were assessed using the Friedman test. For significant changes in oxygenation index and pulmonary mechanics, pairwise comparisons were conducted using the Wilcoxon signed-rank test with p-values adjusted using the Bonferroni correction. Statistical analyses were performed using R.3.6.2 statistical tools, with significance set at an alpha of level 0.05.
The Institutional Review Board approval for this study was granted by the Ethics Committee in Biomedical Research of Cho Ray Hospital (Number 1229/GCN-HĐĐĐ) on November 3, 2021. Written informed consent was obtained from all participants, or their legally authorized representatives. The study adhered to the ethical standards outlined in the Declaration of Helsinki.
3. RESULTS
A total of 46 patients who met the ARDS criteria were enrolled in the study from November 2021 to October 2023 with ARDS classification including moderate ARDS accounted for 76.1% (35 patients) and severe ARDS at 23.9% (11 patients). Table 2 describes the demographics of the study population, which included 32 males (69.6%) and 14 females (30.4%), with a mean age of 49.8 years. The severity scores on admission to the ICU were a SOFA score of 10.7±3.7, and an APACHE II score of 19.0 [IQR 16.3–26.8]. Moreover, the ARDS risk factors included pneumonia accounting for 39.1%, sepsis or septic shock at 37.0%, lung contusion at 8.7%, pancreatitis at 8.7%, and other factors at 6.5%. Respiratory and hemodynamic parameters at ICU admission are shown in Table 2.
Respiratory mechanics were recorded at baseline, 24, and 48 hours, which were compared in Table 3. Patients with ARDS were initially ventilated-guided by the ARDSNet strategy, with a median Pes-exp of 9.7 [IQR 8.7–11.2] cmH2O, and a PL-exp of –0.8 [IQR –1.4–0.78] cmH2O. The median increase in PEEP after modifying the PEEP-guided by transpulmonary pressure was 2 [IQR 2–4] cmH2O, with no significant alterations in hemodynamic parameters, shown in Table 3. There was a substantial improvement in the median PaO2/FiO2 ratio from 135.8 [IQR 100.5–169.8] to 203.6 [IQR 149.0–267.9] at 48 hours (p<0.001), with an absolute difference of 81.5 mmHg between 48 hours and baseline. Similarly, respiratory systems compliance increased from 23.8 [IQR 19.7–27.7] mL/cmH2O to 26.6 [IQR 24.6–30.8] at 24 hours and 26.7 [IQR 22.2–32.2] mL/cmH2O after 48 hours, p<0.001. Moreover, the airway DP showed significant variations during the initial 24 hours, decreasing from a baseline value of 17.0 [IQR 14–20] to 15.0 [IQR: 13–16], p<0.001. Following this initial phase, there were no significant alterations in the airway DP between 24 and 48 hours, shown in Fig. 1. Additional respiratory mechanics characteristics also showed significant changes in Table 4. Procedures-related complications were also noted, with 4 patients (8.7%) experiencing epistaxis which was controlled by local compression, a pneumothorax noted in 1 patient (2.2%), managed with pleural drainage, and there were no occurrences of vomiting or cardiac arrhythmias. Moreover, no patients expired during the study period.
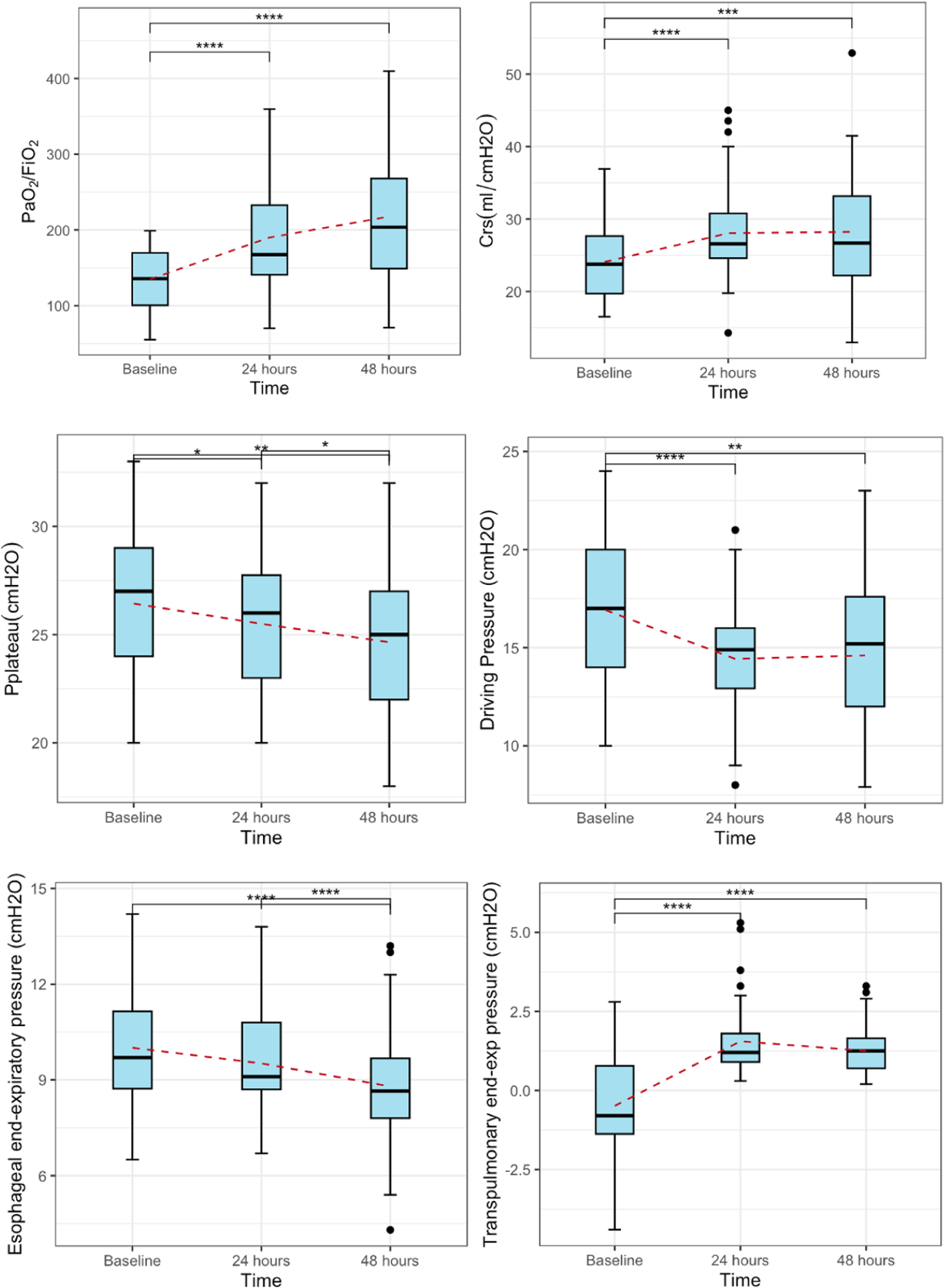
4. DISCUSSION
Mechanical ventilation plays a pivotal role in the management of ARDS. The efficacy of using low tidal volumes in ventilatory strategies has been evidenced [7,8]. However, the clinical benefits of adjusting PEEP remain unclear. Underestimation of setting PEEP may cause potentially over-distended pulmonary alveoli during the expiratory period, resulting in collapse and deterioration. Conversely, excessively high PEEP levels can cause overdistension, right ventricular dysfunction, and hemodynamic compromise [9,10]. To the best of our knowledge, no established PEEP setting approach has been conclusively proven to improve mortality in moderate to severe ARDS patients. Therefore, there is no recommended PEEP setting method in the current guidelines [2]. However, setting PEEP guided by transpulmonary pressure has shown a strong physiological rationale. It has demonstrated clinical outcomes indicating improvements in PaO2/FiO2 ratios and respiratory mechanics parameters compared to empirically set PEEP [11–13]. Furthermore, critically ill patients often manifest increased chest wall weight and elevated basal end-expiratory pleural pressures secondary to edema, effusions, abdominal hypertension, and other causes that may lead to de-recruitment, increased lung elastance, and hypoxemia [4]. Individualized PEEP settings are necessary and selecting a “better PEEP” guided by PL-exp is a more appropriate approach.
The principle of adjusting PEEP guided by Pes was presented by Talmor et al. [12] in the EPVent trial. The trial involved 61 patients and compared titrating PEEP guided by esophageal pressure and titrating PEEP guided low PEEP/FiO2 group. The objective was to change PEEP to PL-exp from negative to positive due to its association with dependent lung collapse [14]. The outcomes indicated a mean of PEEP difference of 6 cmH2O between both groups during the first 72 hours, the Pes-guided group showed a significantly better oxygenation increase of 89 mmHg in PaO2/FiO2 and a 10 mL/cmH2O increase in compliance of respiratory system, along with a trend towards lower mortality in the Pes-guided group (17%) compared to the low PEEP/FiO2 group (39%), p=0.055. This study conducted a comparison between PEEP guided by esophageal pressure and a low PEEP/FiO2 group. This approach results in improvements in blood oxygenation and lung mechanics suggesting the potential for recruitment of collapsed alveoli when setting the table at low PEEP/FiO2. In contrast, Beitler et al. [15] reported the EPVent-2 trial compared a Pes-guided group versus ARDSNet with a high PEEP/FiO2 group, resulting in similar median values of PEEP, transpulmonary pressure at end-inspiration, transpulmonary pressure at end-expiration, plateau pressure, and airway DP in both groups during the first seven days. Therefore, the PaO2/FiO2 ratios, respiratory mechanics parameters, and mortality outcomes showed no significant differences between the two groups. In our study, paralleling the EPVent trial, we initially used low PEEP/FiO2, and all patients had increased PEEP for positive PL-exp. The PaO2/FiO2 ratios and respiratory mechanics parameters within the study group itself, rather than through a direct comparison with a control group. The results showed a significant improvement in blood oxygenation at 48 hours compared to the baseline (p<0.001), as well as in respiratory mechanics such as DP and respiratory system compliance (p<0.001), especially in the first 24-hour period. A negative to positive PL-exp can result in significant effectiveness due to the reduced possibility of pulmonary alveolar collapse. Maintaining the stability of the collapsed alveoli leads to decreased shear force damage during subsequent inspirations which can contribute to enhanced oxygenation. Additionally, effective Protective Lung Ventilation can be achieved by setting PEEP to maintain PL-insp<20 cmH2O within safe limits [4]. Only one patient (2.2%) experienced pneumothorax as a procedure-related complication. However, the limitations of this technique include the necessity of placing an esophageal catheter and accurately positioning the balloon within the esophagus, which presents challenges in implementing this approach. As a final point, our findings support the current recommendation to consider adjusting PEEP-guided by PL-exp as an effective method to improve blood oxygenation and respiratory mechanics in moderate to severe ARDS patients [4,5].
Our study has several limitations. Firstly, it was a single-center study with a small sample size which might limit the generalizability of the findings. Secondly, the absence of a control group for comparison restricts the applicability of our results.
5. CONCLUSION
The study findings suggest that adjusting PEEP guided by transpulmonary pressure led to improvements in oxygenation index and respiratory mechanics. Esophageal manometry emerged as a minimally invasive tool in this regard. These results underscore the need for conducting more targeted research to optimize PEEP settings in ARDS management.