1. INTRODUCTION
The oral cavity, a complex microbial ecosystem, hosts a myriad of microorganisms that play pivotal roles in maintaining a delicate balance between health and disease [1,2]. Individuals using temporary crowns, in particular, are susceptable to various microorganisms, including bacteria, fungi, mycoplasma, viruses, and protozoa. Among these inhabitants, Streptococcus mutans stands out as a major contributor to dental caries, capitalizing on its adeptness at biofilm formation and acidogenicity. Consequently, the most effective approach to preventing oral health issues triggered by S. mutans involves regulating bacterial growth and the formation of biofilm. Understanding the intricate interplay between environmental conditions, nutritional factors, and S. mutans behavior is crucial for unraveling the complexities of dental diseases [3].
The influence of various substances on S. mutans growth and biofilm formation has been a subject of significant scientific inquiry, offering valuable insights into potential strategies for oral health management. Studies [4–6] have explored the impact of various dairy protein types on S. mutans biofilm formation. These investigations suggest that proteins like κ-casein and immunoglobulin G may exert an influence on dental biofilm formation. Additionally, research [7,8] has delved into the role of lactose, the primary sugar in milk, in inducing biofilm formation by S. mutans. The evidence points towards the potential cariogenic effects of lactose, akin to sucrose, emphasizing the significance of understanding how different substances in the oral environment can modulate S. mutans growth and biofilm development [9]. This knowledge is crucial for devising targeted interventions and preventive measures against dental caries and related oral health issues [10].
The profound impact of sugars on S. mutans growth has been extensively documented, shedding light on the intricate relationship between nutritional cues and microbial behavior. Numerous studies have consistently demonstrated that S. mutans, a key player in dental caries initiation, exhibits heightened growth rates in the presence of sugars, particularly sucrose. Studies [7,11,12] underscore the correlation between sucrose abundance and increased biofilm formation by S. mutans. Sucrose serves as a substrate for the production of extracellular polysaccharides (EPS), crucial components of the biofilm matrix, while its fermentation by cariogenic bacteria leads to the production of organic acids. These organic acids, in turn, create an acidic microenvironment that not only supports the acidogenic nature of S. mutans but also contributes to enamel demineralization. The evidence from these studies not only highlights the direct stimulatory effect of sugars on S. mutans growth but also underscores the role of sugars in shaping the microbial biofilm, a critical factor in dental caries development.
Surprisingly, there is a notable dearth of research specifically comparing the effects of different sugars under various conditions on the proliferation and biofilm development of S. mutans. While studies have independently investigated the impact of sugars, such as sucrose and lactose, on S. mutans biofilms [9,13,14], a comprehensive analysis of different sugars under diverse environmental conditions remains missing. Understanding of how sugars, as key nutritional sources, interact with S. mutans in varying atmospheres, including static and shaking conditions, as well as aerobic and anaerobic environments, remains limited. This gap in research hinders a nuanced comprehension of the complex interplay between sugar availability and environmental factors, which are crucial for the modulation of S. mutans growth and biofilm formation. Addressing this research gap would not only contribute substantially to the scientific understanding of oral microbiology but also have practical implications for the development of targeted preventive measures against dental caries. Therefore, in this pursuit, our study presents a comprehensive survey delving into the nuanced conditions influencing S. mutans growth and biofilm formation, with a specific focus on the addition of different sugars under varied culturing conditions. The outcomes of our study hold the potential to inform preventive approaches, fostering innovations in oral health strategies tailored to the dynamic nature of microbial interactions within the oral cavity.
2. MATERIALS AND METHODS
S. mutans ATCC 25175 was employed in this experimental investigation. The strain frozen at –80°C was revived in Brain Heart Infusion broth (BHI, Merck, Germany) overnight at 37°C under aerobic conditions. A bacterial suspension was created utilizing a spectrophotometer (GE Ultrospec 2100 pro, GE Healthcare, Biologix, Piscataway, NJ, USA) to obtain an optical density of OD600=0.1, corresponding to 108 CFU/mL. The suspension was calibrated to McFarland standard 0.01 using BHI supplement. The experiment utilized this bacterial suspension.
The experimental design to study the conditions affecting the growth curve of S. mutans was described in Fig. 1. Three aspects were investigated, including addition of different types of saccharides in culture medium, oxygen concentration and mobility simulation. To examine the effects of sugar addition, the bacterial suspension (1 mL) was diluted with 10 mL of BHI supplemented with different types of saccharides including sucrose, glucose, maltose, fructose, mannitol, D-xylose, D-raffinose pentahydrate and D-mannose, respectively. The concentrations of these saccharides were tested at 1% and 2%. Next, to test the contribution of oxygen level, bacterial suspensions in BHI medium with several sugars were incubated either aerobic or anaerobic at 37°C. To investigate the impact of mobility simulation on S. mutans growth, the bacterial suspensions in BHI medium with several sugars were incubated either with or without shaking at 37°C. All culture experiments were performed in test tubes. Subsequently, the suspensions were homogenized by oscillation. The optical density (OD600) of the aqueous phase was measured to compare the cell growth in the various media used. Each evaluation was performed in triplicate.
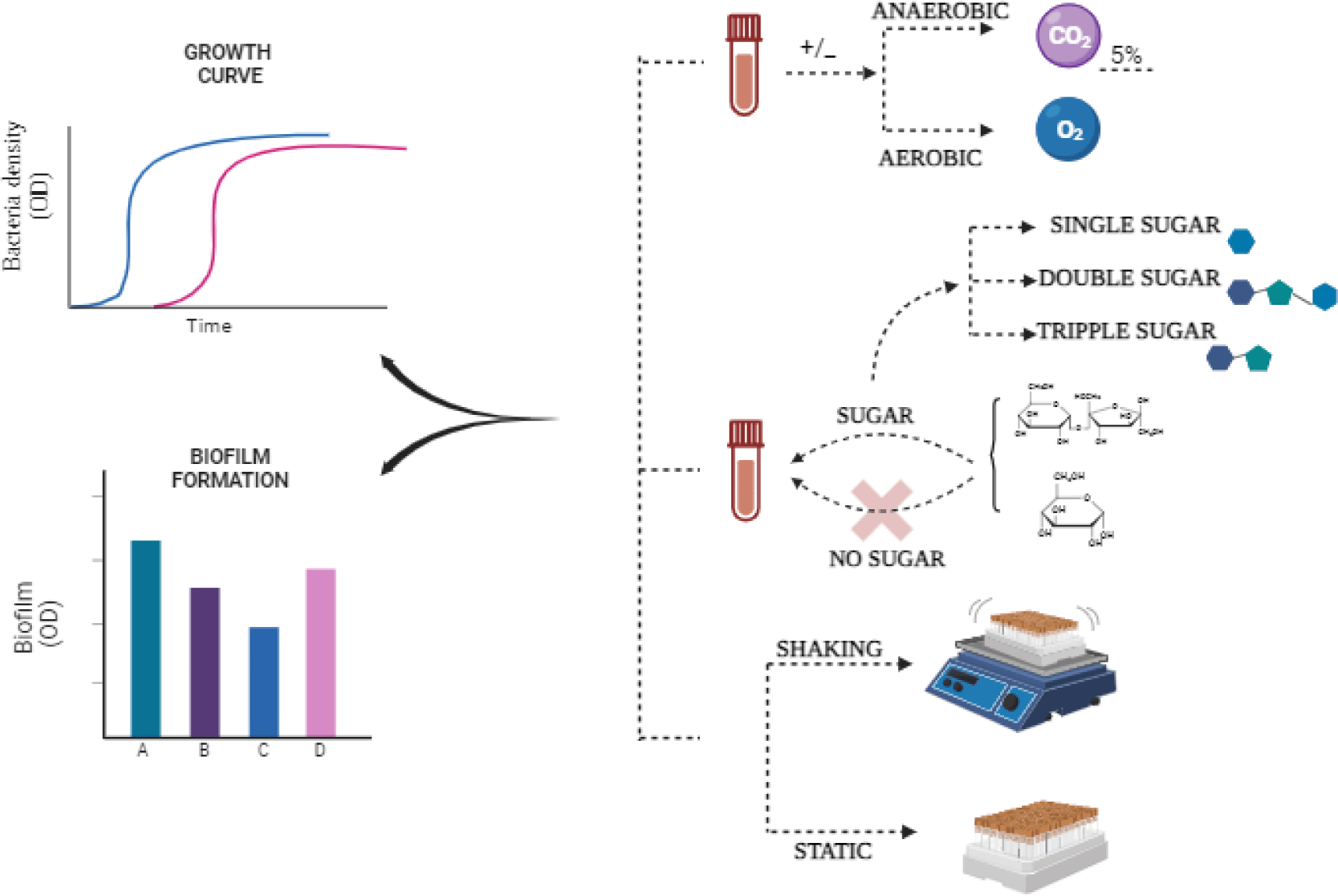
A starting inoculum concentration of 106 CFU/mL was utilized for the establishment of S. mutans biofilm. S. mutans underwent cultivation at 37°C for a duration of 24 hours. Subsequently, the suspension was calibrated to McFarland standard 0.1 in BHI medium. This adjusted suspension was further diluted to attain a concentration of bacterial cells at 106 CFU/mL. All bacterial suspensions were introduced into a 96-well cell culture polystyrene plate (Biologix). In each well, 200 μL of the suspension and BHI medium enriched with varying sugar concentrations were inoculated. The microplates were subjected to aerobic or anaerobic incubation at 37°C for 24 hours. The last row of each polystyrene plate was reserved for controls, with H12 serving as the bacterial growth control. Each evaluation was performed in triplicate.
After a 24-hour incubation period, the free-floating cells were eliminated from each well. The plates underwent three rinses with physiological saline warmed to 37°C. Any surplus moisture was eliminated by gently tapping the microplates on sanitary tissues, followed by a 15-minute drying period in an inverted position within a sterile environment. Subsequently, 200 μL of a 0.1% crystal violet solution (Merck) was introduced into each well. After 30 minutes at room temperature, the crystal violet solution was aspirated, and the microplates were meticulously washed with PBS to eliminate any residual dye. The microplates were subsequently tapped on napkins to eliminate excess liquid and allowed to air-dry. An hour later, 200 μL of absolute alcohol was added to all wells. After a ten-minute interval, a new flat-bottomed microplate containing 200 μL of the previous well contents was prepared for spectrophotometric measurement. The absorbance was recorded at 595 nm using a microplate spectrophotometer (Azure, Palo Alto, CA, USA). The assay was conducted with two replicates for each antibiotic serial dilution.
To ascertain the optimal conditions for growth and biofilm assessment, the absorbance values were subjected to statistical analysis. Significant differences were identified through one-way analysis of variance (ANOVA One-way), employing Tukey’s method for pairwise comparisons. A p-value of 0.05 was established as the threshold for statistical significance. The information is displayed as a value from optical density reflecting biofilm growth. Significant differences in these percentages were determined using a two-way ANOVA, and pairwise comparisons were conducted using Tukey’s method. A significance level of 0.05 for the p-value was deemed statistically meaningful.
3. RESULTS
The growth of S. mutans, a key contributor to oral health issues, is significantly influenced by the addition of various sugars. Given the diverse array of sugars present in daily food intake, the oral cavity provides an ideal environment for S. mutans proliferation. To simulate this oral environment, monosaccharides (fructose, mannose, glucose, D-xylose, and mannitol), disaccharides (maltose, sucrose, and lactose), and trisaccharide (raffinose) were individually introduced into the S. mutans culture medium. Cultures were conducted under both static and shaking conditions, and the growth dynamics were monitored by measuring OD600 at two-hour intervals over 12 hours. Notably, the growth curves of S. mutans exhibited significant changes approximately 4 hours after initiation, indicating the entry into the log phase.
Comparing the planktonic S. mutans OD600 mean values in the presence of monosaccharides under both static and shaking conditions revealed a significant increase compared to the control (Fig. 2A). Interestingly, no significant differences were observed among individual monosaccharides in supporting S. mutans growth. Similar results were obtained when disaccharides (maltose, sucrose, and lactose) were added to the culture medium under both static and shaking conditions (Fig. 2B). Furthermore, the addition of trisaccharide such as raffinose also demonstrated supportive effects on S. mutans growth (Fig. 2C).
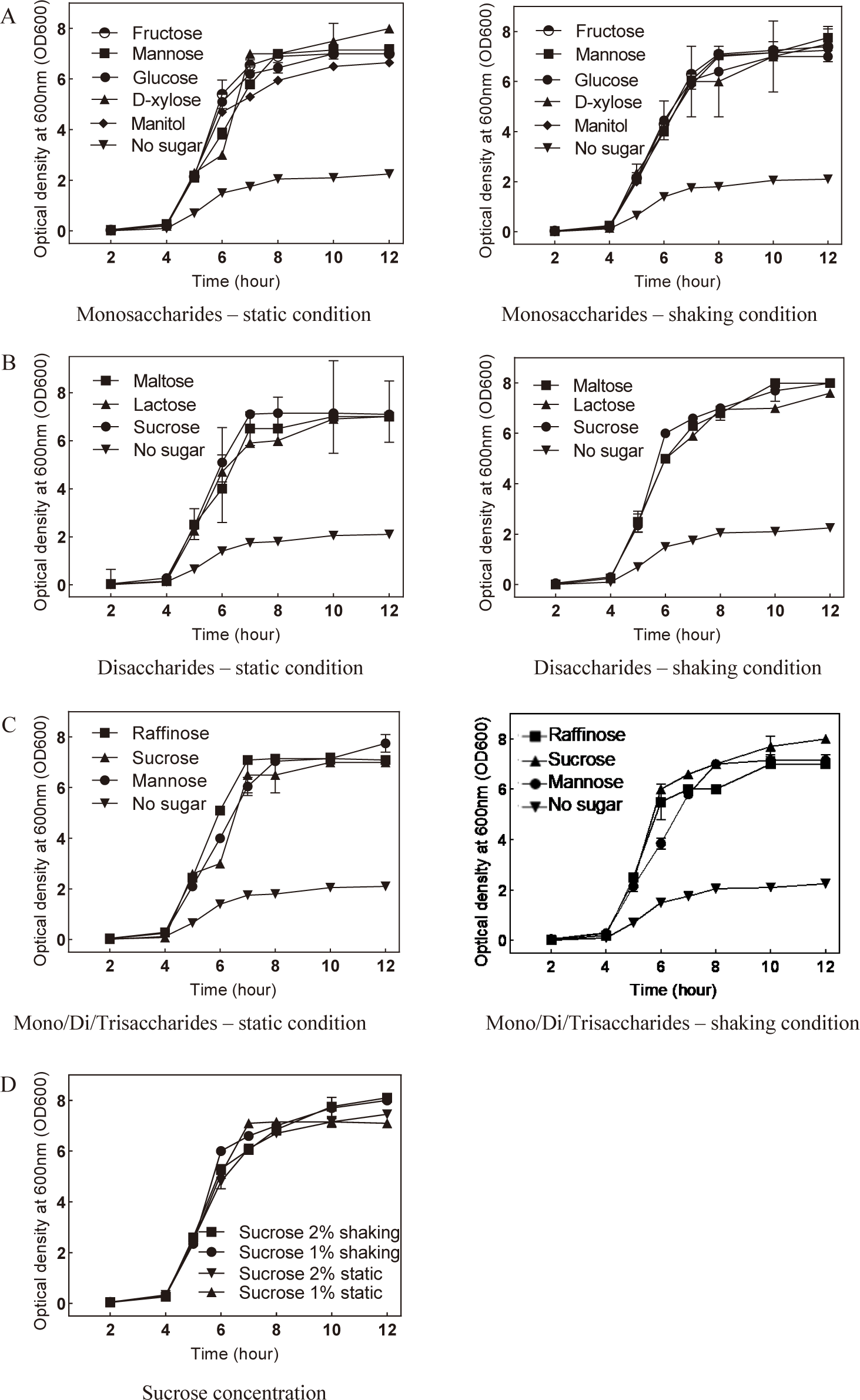
Given that sucrose supplementation exhibited the most pronounced enhancement in S. mutans development, the optimal concentration of sucrose for promoting bacterial growth was determined. Under shaking conditions, the addition of 2% sucrose notably enhanced S. mutans growth compared to other conditions (Fig. 2D). In summary, these findings suggest that the supplementation of 2% sucrose in the culture medium, under conditions mimicking mobility, provides the optimal environment for promoting S. mutans growth.
To investigate the potential benefits of low oxygen levels on S. mutans growth, the bacteria were cultivated in an anaerobic environment with the presence of sugars. The results revealed that culturing S. mutans anaerobically with either monosaccharides or disaccharides enhanced bacterial growth (Fig. 3A and B). Similarly, the incorporation of raffinose under anaerobic conditions also promoted S. mutans growth compared to the control (Fig. 3C). Notably, sucrose supplementation under anaerobic conditions significantly enhanced bacterial growth (Fig. 3B and E). The optimal concentration of sucrose for anaerobic culture was determined to be 2% (Fig. 3D). These findings suggest that sucrose and anaerobic conditions represent advantageous factors that promote the growth of S. mutans.
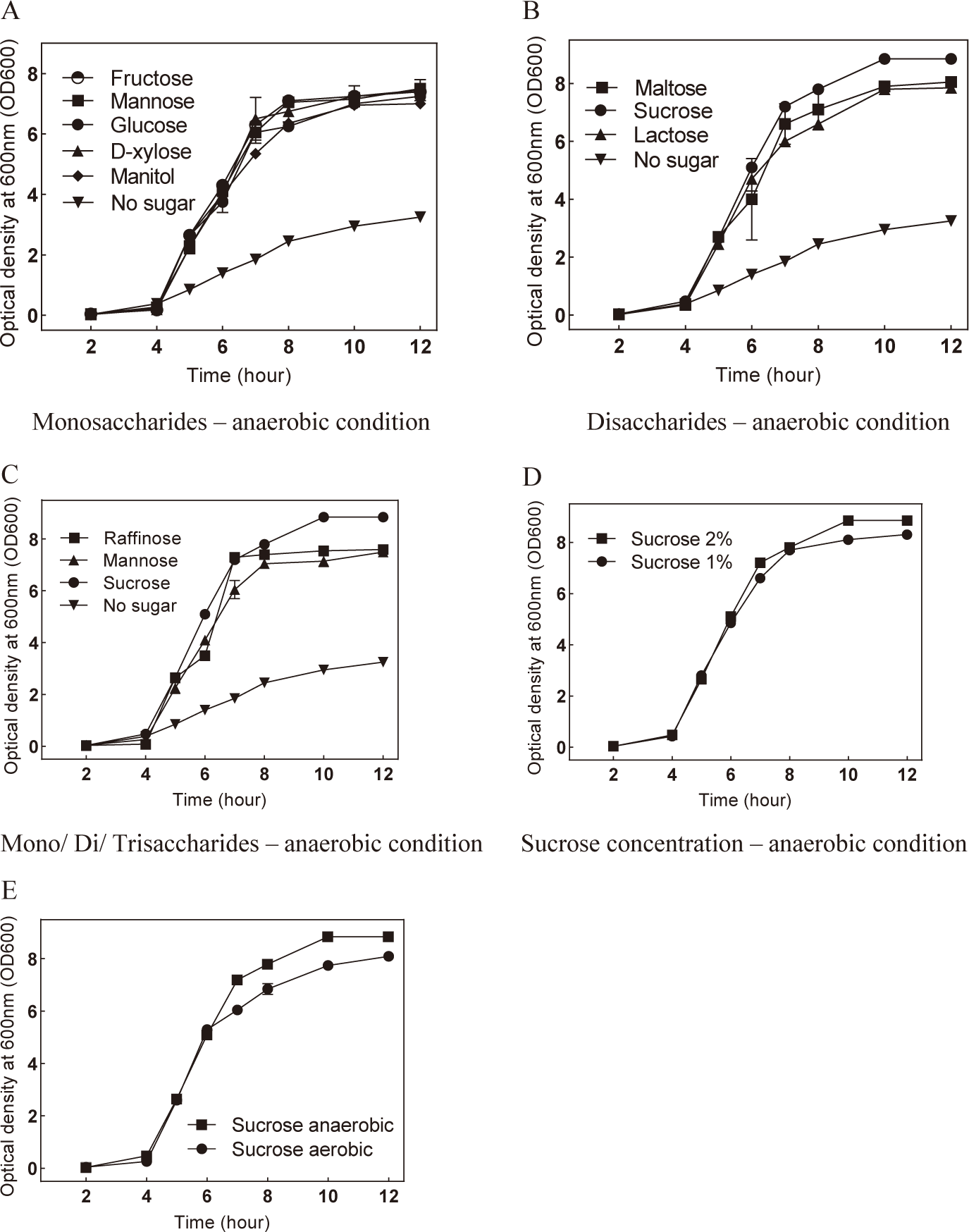
Given that previous findings indicated S. mutans reached the stationary phase at 12 hours post-culture, we sought to validate the conditions conducive to its development by measuring the bacterial cell count at the 12-hour mark using OD600. Under both static and shaking conditions, culturing S. mutans with sugars exhibited substantial promotion in bacterial development after 12 hours (Fig. 4A and B). Among these sugars, the presence of sucrose under shaking conditions demonstrated the most favorable support for bacterial growth. The optimal condition for S. mutans culture was determined to be sucrose at 2% concentration in the presence of mobility simulation (Fig. 4C). Notably, culturing S. mutans with sucrose under anaerobic conditions significantly enhanced bacterial growth compared to aerobic conditions (Fig. 4D and E).
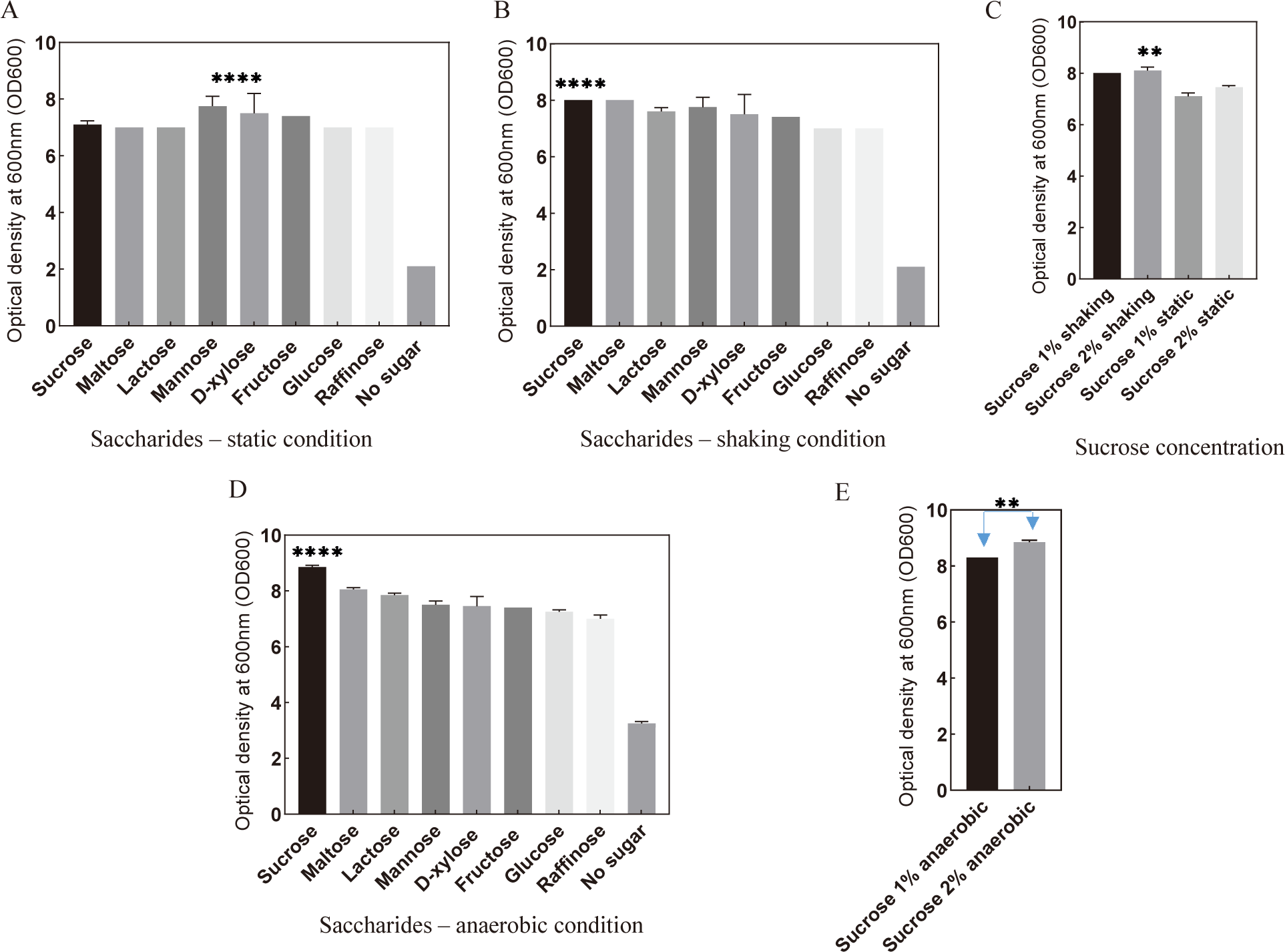
Comparing to the control, the presence of monosaccharides, disaccharides, and trisaccharides showed no significant difference in S. mutans biofilm formation under aerobic conditions (Fig. 5). Nevertheless, the addition of sucrose notably enhanced the biofilm-forming capability of S. mutans (Fig. 5B–D). These findings highlight the crucial role of incorporating 2% sucrose under aerobic conditions for promoting the biofilm formation of the bacteria.
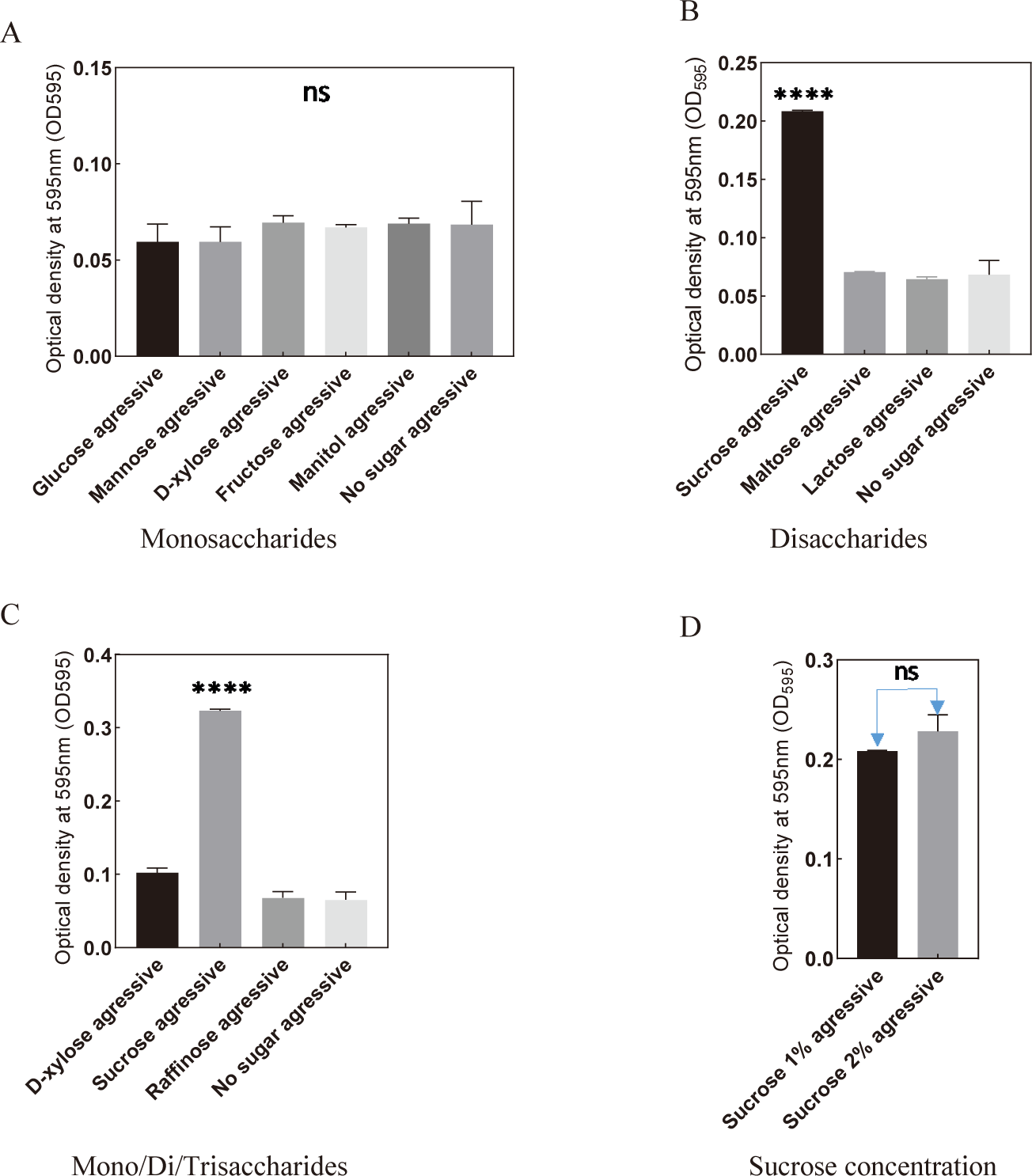
Among various monosaccharides and the control, mannitol exhibited a significant increase in biofilm formation under anaerobic conditions (Fig. 6A). Notably, a substantial enhancement was observed when sucrose was introduced to the culture medium, surpassing the control and the addition of other monosaccharides, disaccharides, or trisaccharides (Fig. 6B and C). Particularly, the addition of 2% sucrose under anaerobic conditions significantly promoted the biofilm-forming ability of S. mutans (Fig. 6D and E).
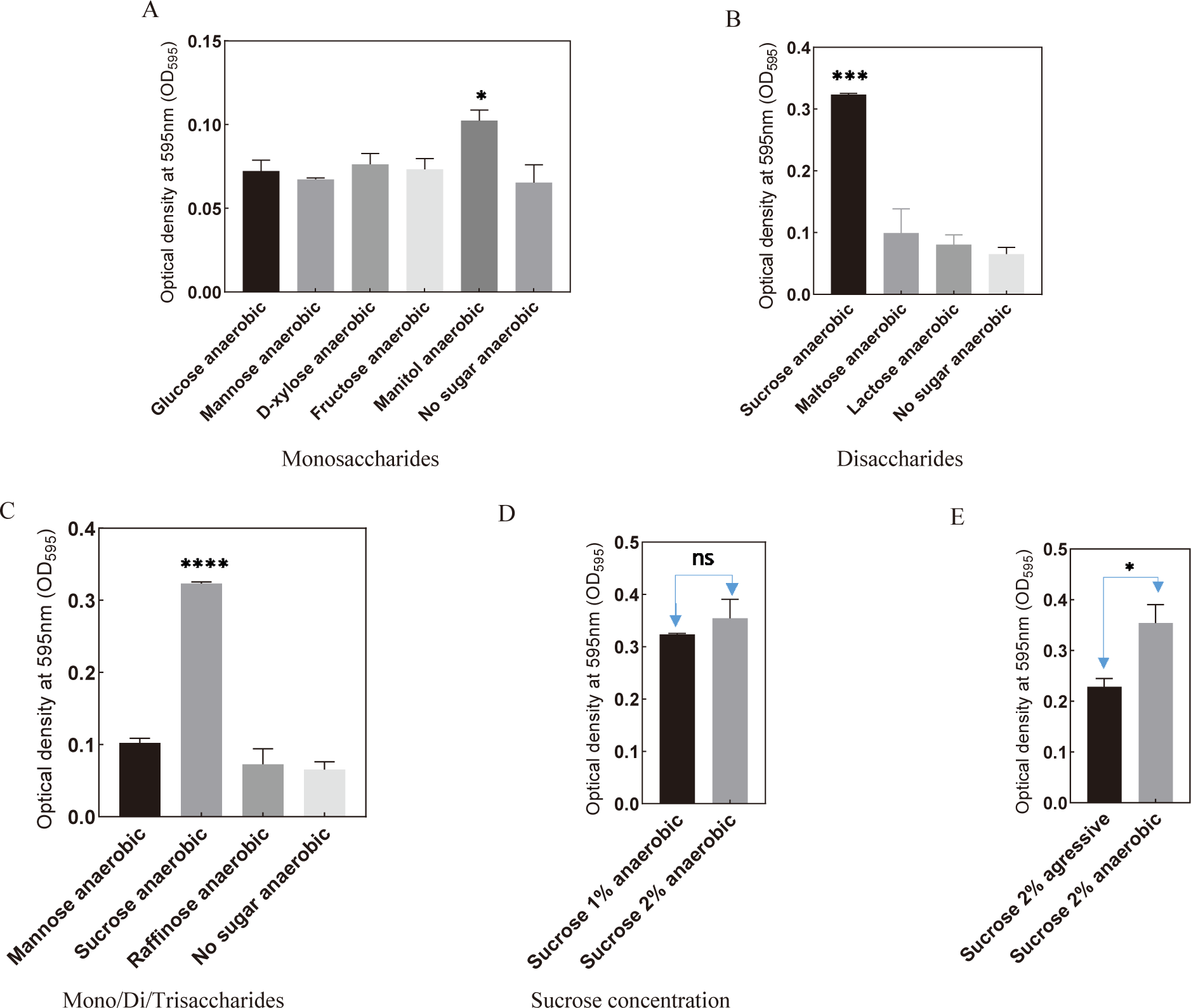
4. DISCUSSION
S. mutans is a significant contributor to dental health, particularly in the development of dental caries or tooth decay. As one of the most prevalent bacteria in the human oral cavity, S. mutans plays a central role in dental plaque formation, a biofilm on tooth surfaces. Through its ability to metabolize dietary sugars and produce acids, S. mutans contributes to enamel demineralization, leading to cavities [15]. Additionally, its capacity to form robust biofilms on tooth surfaces enhances its virulence and persistence in the oral environment, exacerbating dental decay. Biofilm formation by S. mutans involves several key steps (Fig. 7). Initially, adherence to dental surfaces occurs, mediated by sucrose-dependent and sucrose-independent mechanisms. Following attachment, the bacterium begins to produce glucans and fructans, contributing to the formation of a polysaccharide matrix, crucial for biofilm buildup. Interactions with other microorganisms, such as Candida albicans, further enhance glucan production and biofilm formation. S. mutans surface proteins also play a significant role in biofilm development. Overall, these processes facilitate the formation of complex biofilms by S. mutans, contributing to dental caries development and persistence. Our study reveals a noteworthy observation concerning the growth dynamics of S. mutans in response to the addition of various sugars. The consistent promotion of S. mutans growth upon exposure to monosaccharides, disaccharides, or trisaccharides underscores a concerning implication for dental health. In essence, our findings suggest that the consumption of foods containing different types of sugars, regardless of concentration, may contribute to the proliferation of S. mutans, a key contributor to dental caries. This highlights the need for a nuanced understanding of the potential harm associated with diverse sugar sources and concentrations in the context of oral health. The multifaceted impact of sugars on microbial growth in the oral cavity warrants further exploration and consideration in dietary recommendations for maintaining optimal dental well-being.
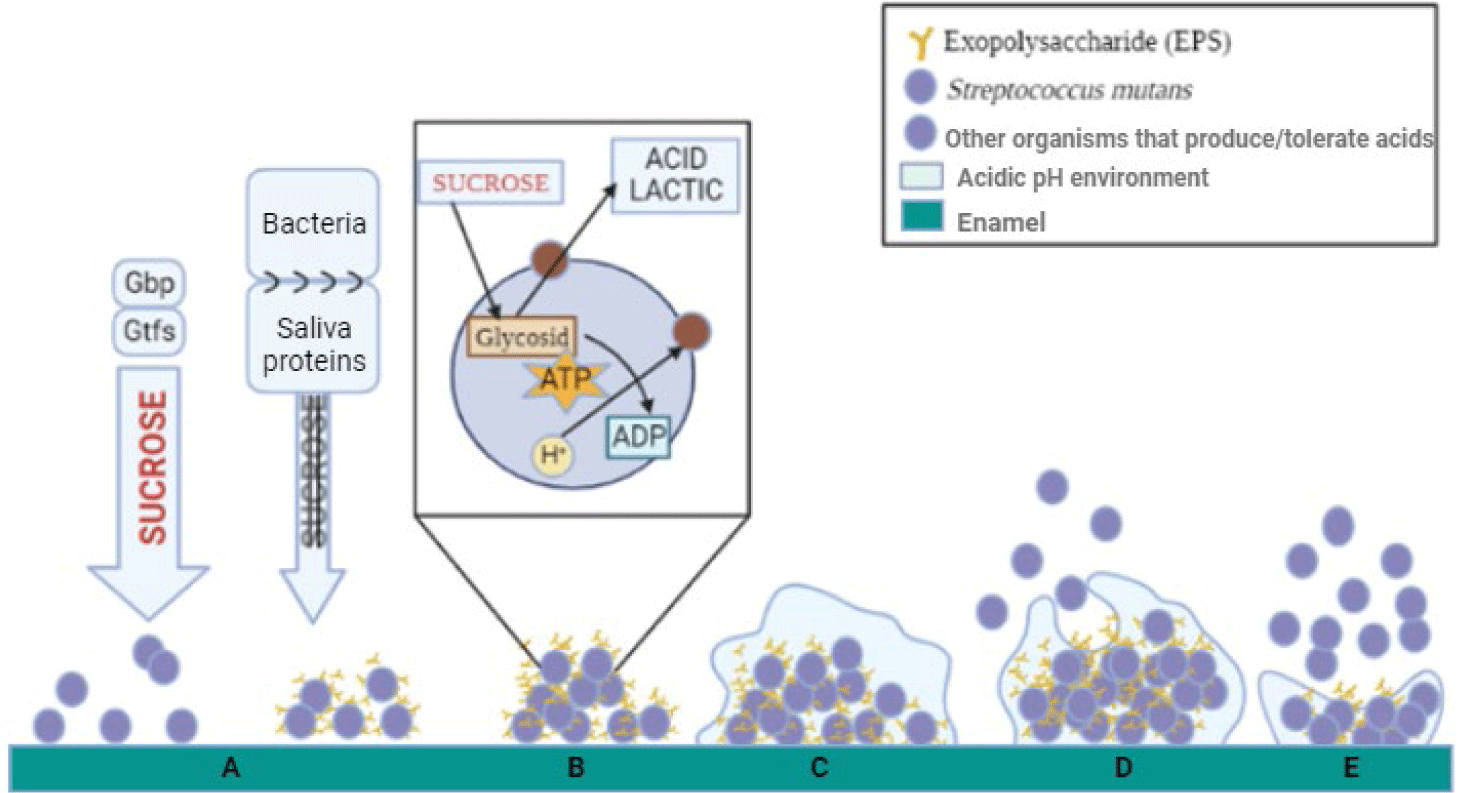
Our investigation sheds light on a crucial aspect of S. mutans behavior by elucidating the impact of sucrose on biofilm formation, a key factor in dental plaque development. The significant support provided by sucrose for S. mutans biofilm formation aligns with its well-established role as a primary contributor to tooth plaque. This observation gains further credibility when considering the robust the formation of biofilm by S. mutans when exposed to sucrose under anaerobic conditions, a finding that resonates with the biological characteristics of S. mutans. Our study suggests that the gingival sulcus, the space between the tooth surface and the sulcular epithelium, serves as an optimal site for S. mutans biofilm formation, particularly when sucrose is present in the oral cavity. This corroborates existing knowledge about the propensity of S. mutans to thrive in specific oral niches, emphasizing the importance of understanding the environmental cues influencing biofilm development for targeted preventive strategies.
Our investigation underscores the widespread presence of sucrose in both natural and processed foods, with significant implications for S. mutans biofilm formation and,its impact on dental health. Sucrose, a prevalent disaccharide, naturally occurs in a diverse array of dietary items, encompassing specific fruits, vegetables, starches, various nuts, and a multitude of sweeteners. Furthermore, processed foods, which are frequently consumed in modern diets, are often enriched with sucrose through the addition of flour and/or added sugars. Notably, most of these food items inherently contain sucrose concentrations exceeding 2% [16–18]. Our results align with this natural prevalence, demonstrating that a 2% sucrose concentration optimally supports S. mutans biofilm formation. This link between dietary sucrose levels and microbial biofilm formation highlights the potential influence of everyday dietary habits on oral health and stresses the importance of investigating preventive strategies in dental care.
Our investigation into the growth dynamics of S. mutans under both static and shaking conditions revealed a noteworthy observation—the absence of a significant difference in the growth curve between the two conditions. This implies that the static condition, which involves a more stable and unagitated environment, is just as conducive to the growth of S. mutans as the dynamic, shaking condition. The lack of a substantial variation suggests that the static condition may offer specific advantages for future research focused on S. mutans. Importantly, the static condition better mimics the environment within the oral cavity, where conditions are relatively stable. This finding holds important implications for experimental design, demonstrating that static conditions can serve as a representative model for studying oral environments and can provide valuable insights into S. mutans growth dynamics without the confounding effects of mechanical agitation.
5. CONCLUSION
The oral cavity provides an ideal environment for the growth and development of various bacteria. S. mutans, a significant contributor to tooth decay, particularly in individuals with dental temporary crowns, thrives in this setting. To replicate the conditions within the oral cavity for those using dental temporary materials, we explored several variables to determine the optimal environment for the S. mutans growth and biofilm on polystyrene plates. Our findings demonstrate that cultivating S. mutans in the presence of a 2% sucrose solution under anaerobic conditions with mobility simulation is higly effectove in promoting both growth and biofilm formation on polystyrene surfaces. This discovery offers valuable insights for improving culture condition for future research in dental microbiology.